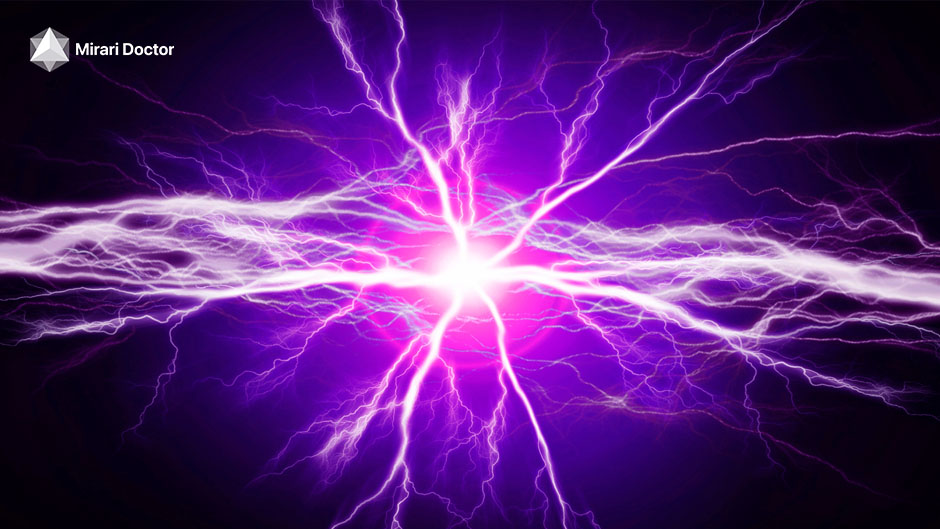
You May Be Interested In:
Cold plasma and hot plasma, the two primary states of plasma, present a fascinating dichotomy in physics and engineering applications. Cold plasma operates at much lower temperatures compared to its hot counterpart, comprising non-equilibrium states where energetic electrons drive chemical processes, leaving the bulk gas temperature relatively low. In contrast, hot plasma involves high temperatures, often reaching millions of degrees, found naturally in stars and artificially in fusion reactors. Understanding these plasma states is crucial as it opens up diverse practical applications, from environmental remediation and medical therapies for cold plasma to high-energy applications such as welding and nuclear fusion for hot plasma. In this comprehensive exploration, we delve into their physical characteristics, production methods, applications, mechanisms, and benefits, providing insights into their distinct behaviors and future potentials.
One notable development in the field of cold plasma is the Mirari Cold Plasma device, created by General Vibronics. This handheld device utilizes innovative technology to harness the power of nitric oxide (NO) for generating a unique form of non-invasive cold plasma. With approvals from the Thai FDA and Vietnam MOH for specific uses, the Mirari Cold Plasma System showcases the growing potential and real-world applications of cold plasma technology, especially in the medical sector.
Physical characteristics
Temperature differences
In the realm of plasma, temperature delineates the boundary between cold and hot variants. Cold plasma, often called nonthermal plasma, is akin to a chilled breeze on a summer day; it’s invigorating without searing one’s skin. Despite having electron temperatures that can soar to several thousand degrees, the ions and neutral particles remain mild, typically close to room temperature. Research by Laroussi et al. (2005) illustrated that the temperature of ions and neutrals in cold plasma hovers around 300K, making it invaluable for applications sensitive to heat, like surface treatments or medical therapies where delicate tissues could be compromised by higher temperatures.
Hot plasma, on the other hand, is like the blazing core of a furnace. Here, both electrons and ions attain staggering temperatures, often exceeding thousands of degrees Celsius (up to 10 million degrees in nuclear fusion contexts), achieving a state of local thermodynamic equilibrium (LTE). This thermal balance, examined extensively in studies like those by Chen (2016), means all particles share energy uniformly, making hot plasmas indispensable in high-energy contexts such as industrial material processing or astrophysical research.
Table: Temperature Comparison between Cold and Hot Plasma
Characteristics | Cold Plasma | Hot Plasma |
---|---|---|
Electron Temperature | Thousands of degrees (K) | Thousands to millions of degrees (K) |
Ion Temperature | Close to room temperature (300K) | Thousands to millions of degrees (K) |
Thermal Equilibrium | Non-equilibrium | Local thermodynamic equilibrium |
Common Applications | Surface treatments, medical therapies | Industrial processing, nuclear fusion |
The low temperatures of cold plasma enable its use in temperature-sensitive applications, such as the Mirari Cold Plasma device. By maintaining bulk gas temperatures close to room temperature while still generating reactive species, this technology can be safely applied for various medical purposes without causing thermal damage to biological tissues.
The differing temperature dynamics underscore distinct applicational avenues. The achingly high ion temperatures of hot plasma foster environments suitable for material synthesis or nuclear reactions, where substantial energy inputs are essential. Conversely, the cool serenity of cold plasma allows gentle yet effective utilizations like sterilization processes that don’t scorch the substrate, providing a serene but mightily effective touch.
Ionization degree
Ionization degree, the measure of charged particles (ions and electrons) to the total number of particles, marks another key characteristic distinguishing cold from hot plasma. Cold plasma, typically weakly ionized, has an ionization degree often less than 1%, sometimes dipping to 10^-4. This lower ionization, akin to a sprinkle of spice in a large pot of soup, introduces significant reactivity without overwhelming the system with energy.
Research indicates practical applications favoring low ionization degrees in standard ion sources, around 10^-4 to 10^-5, serving contexts that benefit from precision and control. For instance, in biomedical applications, cold plasma provides antimicrobial actions through reactive species without the intense energy that could damage tissues, as highlighted by studies from Laroussi (2002).
Hot plasmas contrast strikingly, often being nearly fully ionized, with degrees of ionization approaching 100%. Here, every particle, charged fervently, contributes to a seething cauldron of energy. This high ionization degree provides the raw power necessary for processes like plasma arc welding, where robust energy is pivotal for cutting through tough materials or generating the immense pressures needed for nuclear fusion.
Bullet Points: Ionization Degree Difference
- Cold Plasma:
- Ionization degree less than 1%
- Attributes like a gentle breeze, suitable for delicate applications
- Utilized in environments where minimal thermal impact is crucial, such as in medical sterilization and surface modification
- Hot Plasma:
- Nearly 100% ionization
- Resembles a roaring fire, fueling high-energy reactions
- Employed in industrial and research applications requiring substantial energy inputs like fusion reactors and advanced material processing
The low ionization degree of cold plasma makes it well-suited for technologies like the Mirari Cold Plasma device. By generating a sufficient amount of reactive species to achieve desired effects while keeping the overall ionization low, cold plasma can be applied safely and effectively in medical contexts without causing collateral damage.
The stark contrast in ionization degrees plays a decisive role in the applicability of cold versus hot plasma, ensuring that each serves its niche effectively. As a result, industries ranging from healthcare to aerospace can harness these plasma states to meet diverse, precise needs.
Density variations
Density, the number of particles per unit volume, varies notably between cold and hot plasma, influencing their behaviors and applicability. Cold plasma, typically found in lower densities, reflects a sparse constellation of reactive particles scattered lightly through space. The neutral gas densities in cold plasmas, reported to be around 10^13 to 10^16 molecules/cm^3 at pressures ranging from ~0.1 to 10^3 Pa, highlight this diluted yet potent state. Such conditions ensure reactive species can interact widely with substrates or biological matter without overwhelming energy concentration.
In contrast, hot plasmas often feature higher densities consistent with the seething core of a star or the intense conditions of a fusion reactor. These environments, marked by high-energy particle collisions and dense particle packing, facilitate powerful interactions that drive processes such as nuclear fusion or high-temperature material cutting.
Table: Density Variations in Cold and Hot Plasma
Characteristics | Cold Plasma | Hot Plasma |
---|---|---|
Particle Density | 10^13 to 10^16 molecules/cm^3 | Higher densities in densely packed states |
Pressure Range | ~0.1 to 10^3 Pa | High pressures in controlled environments |
Interaction Mode | Sparse, reactive interactions | Intense, high-energy collisions |
Common Examples | Ionosphere, surface treatments | Stars, fusion reactors |
The Mirari Cold Plasma device operates within the typical density range of cold plasmas. This allows it to generate a sufficient concentration of reactive species to achieve therapeutic effects while maintaining a safe and controlled environment. The low particle density ensures that the energy is distributed evenly, preventing localized hot spots that could cause unintended damage.
The density variations impart unique qualities to cold and hot plasmas. Cold plasmas, with their lower density, offer controlled reactivity akin to a precision scalpel in surgery ensuring that only specific interactions occur, beneficial for sensitive applications such as surface treatments and precision cleaning. Hot plasmas, exemplifying a sledgehammer approach, embody a realm where intense kinetic interactions foster fusion research, pushing the boundaries of energy applications.
The intrinsic properties of cold and hot plasma, defined by temperature, ionization degree, and density, frame their diverse applicational potential, making each a pivotal player across various technological frontiers.
Production methods
Generation of cold plasma
Cold plasma, or nonthermal plasma, is produced through methods that maintain low temperatures yet foster significant reactivity. This process resembles the artistry of a glassblower shaping delicate structures with precise, controlled heat. Here are the primary methods:
- Dielectric Barrier Discharge (DBD): Involves high voltage across electrodes separated by a dielectric barrier. It ionizes the gas, generating plasma at around room temperature. This method is widely used for sterilization and surface treatment, akin to a painter adding delicate details to a canvas without altering the underlying material’s form. The Mirari Cold Plasma device employs a unique form of DBD to generate its therapeutic cold plasma.
- Gliding Arc Discharge: Creates plasma using an electric arc that glides along an electrode’s surface, keeping temperatures low while generating reactive species. Ideal for waste treatment, this method ensures that the reactive species can dismantle contaminants like a precise chemical scalpel.
- Plasma Jet: Produces plasma by passing gas through a high-frequency electric field, creating a focused plasma stream. This method, used in biomedical treatments, allows for targeted, precise applications akin to a skilled surgeon’s scalpel.
- Microwave Plasma: Utilizes microwaves to excite gas molecules, generating plasma at low pressures. Effective for synthesizing nanomaterials, this method works like a microwave heating food uniformly, ensuring thorough processing without burning.
- Penning Discharge: Relies on electron interactions with gas atoms, sustaining a discharge to produce plasma. Used in ion sources and photon generation, this method parallels a controlled flash of lightning, precise and intensely reactive.
Bullet Points: Production Methods of Cold Plasma
- DBD: High voltage ionization across dielectric barriers – used in surface treatment and sterilization. Employed by the Mirari Cold Plasma device.
- Gliding Arc: Electric arc movement generates reactive species – ideal for chemical synthesis and waste treatment.
- Plasma Jet: Gas passed through high-frequency fields – utilized in biomedical surface treatments.
- Microwave Plasma: Gas excitation via microwaves at low pressures – effective in nanomaterials synthesis.
- Penning Discharge: Electron-gas atom interactions – relevant for ion sources and photon production.
These methods underscore the versatility and precision of cold plasma generation, facilitating applications across realms requiring low thermal impact and high reactivity. The Mirari Cold Plasma device’s innovative DBD technology showcases how these principles can be harnessed for groundbreaking medical applications.
Methods for hot plasma production
Hot plasma, or thermal plasma, demands methods that inject substantial energy, akin to the rigorous forging process of a blacksmith shaping metal. The main production methods include:
- DC Arc Discharges: Utilizes direct current to generate a spark between electrodes, ionizing the gas and creating a stable arc. This high-temperature approach, reaching several thousand degrees Celsius, is instrumental in processes like welding and material vaporization.
- Plasma Torches: Convert electrical energy into high-temperature plasma jets using compressed gases. Frequently employed in cutting and welding, a plasma torch ensures precise cuts through hard materials, much like a diamond-tipped blade.
- Radiofrequency (RF) Induction Heating: RF coils generate an alternating electric field to ionize gas, maintaining hot plasma. Used in material processing, this method is akin to a sophisticated, evenly distributed heat ensuring uniform material treatment.
- Microwave Plasma: Microwaves ionize gas to create plasma, transferring energy efficiently to electrons. Essential in high-intensity applications, this method parallels food cooked quickly yet thoroughly in a microwave oven.
- Laser-Induced Plasma: High-intensity laser beams ionize gas, generating localized, controlled plasma, suitable for specialized tasks such as material science and medical technologies.
Table: Methods for Hot Plasma Production
Method | Description | Common Applications |
---|---|---|
DC Arc Discharges | Direct current sparks between electrodes | Welding, material vaporization |
Plasma Torches | Electrical energy into high-temperature plasma jets | Cutting, welding |
RF Induction Heating | RF coils generate alternating electric fields | Material processing |
Microwave Plasma | Microwaves ionize gas, creating efficient plasma | High-intensity applications |
Laser-Induced Plasma | High-intensity lasers ionize gas | Material science, medical technologies |
Hot plasma’s robust production methods provide the immense energy required for demanding industrial and fuel-generating reactions, mirroring the transformative power of a furnace.
Equipment and technologies used
For both cold and hot plasma production, specialized equipment and modern technologies are pivotal. Tools and technologies differ significantly, reflecting the energy needs and operational conditions unique to each plasma state.
- Cold Plasma Equipment:
- DBD Reactors: Compact reactors for surface treatment and sterilization. The Mirari Cold Plasma device is an example of a portable DBD reactor designed for medical applications.
- Plasma Jets: Handheld jet devices for medical and precision applications.
- Microwave Generators: Devices to produce cold microwave plasma at low pressures.
- Atmospheric Pressure Plasma Devices: Used for low-temperature plasma generation under atmospheric conditions.
- Penning Discharge Chambers: Devices for creating controlled low-temperature plasmas for ion source applications.
- Hot Plasma Equipment:
- DC Power Supplies: For initiating and sustaining DC arc discharges.
- Plasma Torches: Industrial-grade torches for cutting, welding, and material processing.
- RF Generators: Induction coils for maintaining high-temperature RF plasmas.
- Microwave Generators: High-power devices creating intense microwave plasmas.
- Laser Systems: High-intensity laser setups for inducing hot plasmas in specialized applications.
Bullet Points: Equipment and Technologies
- Cold Plasma Equipment:
- DBD Reactors: Surface treatment, sterilization. Mirari Cold Plasma device is a portable DBD reactor.
- Plasma Jets: Precision medical treatments.
- Microwave Generators: Nanomaterial synthesis.
- Atmospheric Plasma Devices: Versatile low-temperature plasma generation.
- Penning Discharge Chambers: Ion sources and photon generation.
- Hot Plasma Equipment:
- DC Power Supplies: Sustaining arc discharges.
- Plasma Torches: Cutting, welding.
- RF Generators: High-temperature material processing.
- Microwave Generators: Intense plasma creation.
- Laser Systems: Material science and medical applications.
This spectrum of equipment underscores the tailored needs of cold and hot plasma, aligning precise tools with their respective operational contexts to effectively harness plasma’s transformative potential. Innovations like the Mirari Cold Plasma device demonstrate how these technologies can be made more accessible and practical for real-world uses.
Applications
Biomedical applications of cold plasma
Cold plasma’s gentleness and precision have unlocked revolutionary biomedical applications. Imagine a gentle caress, soothing yet powerfully transformative. Here are the key biomedical applications:
- Wound Healing: Cold atmospheric plasma accelerates healing in chronic and acute wounds. By reducing bacterial load and promoting tissue regeneration, it operates as a microbial assassin, fostering conditions wherein recalcitrant wounds, like diabetic ulcers, mend more effectively. Early clinical trials underscore its efficacy in reducing infections and accelerating healing. The Mirari Cold Plasma device has shown promise in this area, offering a portable and non-invasive treatment option.
- Sterilization: Exhibiting formidable sterilizing prowess, cold plasma can neutralize an array of pathogens, including resilient strains like MRSA, playing an indispensable role in infection control within healthcare settings, akin to a vigilant guardian shielding against unseen microbial foes.
- Cancer Treatment: In oncology, cold plasma selectively targets cancer cells, inducing apoptosis through reactive oxygen species (ROS). This selective lethality spearheads its potential as an adjunct therapy in cancer treatment, promising a future where plasma might play a pivotal role in cancer eradication. The Mirari Cold Plasma System’s ability to generate nitric oxide (NO) could potentially be harnessed for this application.
- Dental Applications: Cold plasma’s antibacterial properties are capitalized upon in dentistry. Procedures such as root canal treatments and tool sterilization benefit from plasma’s ability to decimate biofilms, enhancing infection control and ensuring safer dental procedures.
- Tissue Engineering: Cold plasma’s knack for enhancing cell adhesion and proliferation marks it valuable in tissue engineering. It modifies biomaterial surfaces, promoting better tissue integration and spurring regenerative processes akin to a catalyst in a delicate symbiosis.
Table: Biomedical Applications of Cold Plasma
Application | Description | Benefits |
---|---|---|
Wound Healing | Promotes healing, reduces infections | Accelerates tissue regeneration |
Sterilization | Neutralizes pathogens, including MRSA | Enhances hospital hygiene |
Cancer Treatment | Selectively induces cancer cell death | Potential adjunct cancer therapy |
Dental Applications | Eliminates dental biofilms | Improves infection control |
Tissue Engineering | Enhances cell adhesion and proliferation | Advances regenerative medicine |
The Mirari Cold Plasma device’s unique nitric oxide generation capabilities position it as a promising tool across these biomedical applications. Its portability and non-invasive nature make it particularly well-suited for wound healing and infection control purposes. As research continues to uncover the full potential of cold plasma and nitric oxide in medicine, devices like Mirari are poised to play an increasingly important role.
Cold plasma’s unique attributes translate into groundbreaking advancements in biomedical fields, offering healing touch coupled with potent effectiveness.
Industrial uses of hot plasma
Hot plasma, embodying raw, unbridled energy, sees extensive use in industrial applications. Its blazing intensity parallels the forceful strikes of a blacksmith’s hammer, shaping materials under tremendous heat and pressure.
- Fusion Research: Hot plasma forms the cornerstone of nuclear fusion experiments. Temperatures in the millions of degrees Celsius, achieved in devices such as tokamaks, replicate stellar conditions necessary for fusion, illuminating pathways to potentially limitless clean energy.
- Plasma Cutting: Utilizing high-energy plasma jets, this technique slices through metals with precision, akin to a master jeweler crafting intricate designs. The intense heat ensures minimal distortion, proving invaluable in industrial fabrication and repair.
- Material Processing: Processes like plasma spraying deposit molten material coatings, enhancing surface properties. Chemical vapor deposition (CVD) employs hot plasma to produce thin films with specific characteristics, vital in semiconductor manufacturing and advanced materials science.
- Waste Treatment: Hot plasma can disintegrate hazardous waste, reducing toxic substances through high-energy reactions. This method transforms noxious materials into benign compounds, offering an eco-friendly solution to industrial waste management.
Bullet Points: Industrial Uses of Hot Plasma
- Fusion Research: Essential in devices like tokamaks for replicating fusion conditions.
- Plasma Cutting: Precision cutting of metals with minimal distortion.
- Material Processing: Includes plasma spraying and CVD for enhancing surface properties.
- Waste Treatment: Breaks down hazardous materials into non-toxic compounds.
The high-temperature environment of hot plasma is pivotal in industries requiring robust, high-energy processes, delivering unrivaled effectiveness across a spectrum of demanding applications.
Environmental applications of cold plasma
Cold plasma’s reactivity at low temperatures makes it an environmental superhero, combating pollution and promoting sustainability without the scorched earth approach of hotter methods.
- Water Treatment: Cold plasma technology effectively removes pollutants like organic compounds and microorganisms from water, achieving purity through advanced oxidation processes, akin to a purifier offering the elixir of cleanliness. The Mirari Cold Plasma device’s nitric oxide generation could potentially be explored for this purpose.
- Air Purification: Capable of degrading volatile organic compounds (VOCs) and other contaminants, cold plasma provides an eco-friendly solution to air quality improvement, much like a breath of fresh air amidst urban smog. The portable nature of devices like Mirari Cold Plasma System could make them useful for localized air purification needs.
- Soil Remediation: It breaks down hazardous substances in soil, ameliorating pollution, and rejuvenating soil health. This sustainable approach ensures a fertile future, mirroring nature’s resilience.
- Food Safety: Cold plasma inactivates microorganisms and degrades toxins in food processing, ensuring safety and extending shelf life without the need for thermal processing, akin to a gentle guardian maintaining food integrity. The Mirari Cold Plasma device’s approval for specific uses demonstrates its potential in this domain.
Table: Environmental Applications of Cold Plasma
Application | Description | Benefits |
---|---|---|
Water Treatment | Removes pollutants and microorganisms | Achieves high purity |
Air Purification | Degrades VOCs and contaminants | Improves air quality |
Soil Remediation | Breaks down hazardous substances | Restores soil health |
Food Safety | Inactivates microorganisms in food processing | Enhances safety, extends shelf life |
The Mirari Cold Plasma device, with its innovative nitric oxide generation and portability, presents exciting possibilities for environmental applications. While more research is needed to fully understand its capabilities in these areas, the device’s proven efficacy in specific domains hints at its broader potential.
Cold plasma’s environmentally benign capabilities emphasize its role in sustainable solutions, ensuring the health of ecosystems while maintaining efficacy in pollution control.
Mechanisms and behavior
Thermal and non-thermal dynamics
Thermal and non-thermal plasmas, differentiated by their energy dynamics, epitomize the unique natures of hot and cold plasmas. Thermal plasma, like the intense heat of a forge, involves high-energy states where both electrons and positive ions maintain significant energy equivalence. This equilibrium generates a plasma where the distribution of particle energies follows a Maxwellian pattern, indicative of heat balance across particles.
Cold plasma, on the other hand, operates akin to a cohesive orchestra, where the electrons are energetic maestros driving reactions, while ions and neutrals remain in a non-thermal, relatively cooler state. This lack of equilibrium allows for the generation of reactive species necessary for applications like sterilization or surface treatment without inducing bulk thermal damage the particles are high in energy yet disparate in their thermal states.
Research by Lieberman and Lichtenberg (2005) elucidates that cold plasma generation methods, like Dielectric Barrier Discharge (DBD) or glow discharge, produce diverse reactive particles through interactions that are disproportionately driven by electron energies. These reactive species, including free radicals and ions, can interact withsurfaces and substrates in ways that promote significant chemical changes without the associated heat damages common in thermal plasma processes. The Mirari Cold Plasma device capitalizes on these non-thermal dynamics to generate therapeutic nitric oxide without causing thermal damage to tissues.
Comparison and Mechanistic Insights:
- Hot Plasma (Thermal Dynamics):
- Energy Distribution: Symmetric Maxwellian distribution, where energy is dispersed uniformly among particles, reflecting thermal equilibrium.
- Mechanisms: Utilizes intense electromagnetic fields to sustain high energy levels, facilitating robust kinetic interactions necessary for high-energy processes like fusion and welding.
- Behavior: High reactivity sustained by significant intermolecular collisions, leading to uniformly high temperatures and broad particle ionization.
- Applications: Primarily in environments needing substantial energy inputs, such as plasma arcs and fusion reactors.
- Cold Plasma (Non-Thermal Dynamics):
- Energy Distribution: Asymmetric, with high-energy electrons but cooler ions and neutrals, creating a non-equilibrium state.
- Mechanisms: Generated through DBD, microwave discharge, and other methods emphasizing electron energy while maintaining low thermal impacts on the bulk medium. The Mirari Cold Plasma device uses a unique DBD mechanism to generate nitric oxide.
- Behavior: High electron-driven reactivity with minimal heat transfer to surrounding materials, promoting delicate surface modifications and microbial inactivations.
- Applications: Found in sectors requiring low thermal load, such as medical therapies, food safety, and material processing.
Electron and ion temperature discrepancy
In plasma, electron and ion temperature discrepancies are influenced by several factors, reflecting the intricate dance of energy exchange and collisions. This temperature variance is pivotal in defining the behavior and applications of both cold and hot plasma environments.
- Differential Collisional Rates: In plasmas, electrons, being significantly lighter than ions, collide more frequently and involve high-energy processes, leading to higher temperatures. Conversely, the heavier ions experience fewer collisions, which translates to lower energy states and thermal differentials. This phenomenon is especially prominent in non-thermal plasmas (cold plasma), where high-velocity electrons drive the reactive process while ions and neutral particles remain at much cooler temperatures. The Mirari Cold Plasma device utilizes this differential collisional behavior to generate reactive nitric oxide species without heating the bulk gas.
- Energy Transfer Mechanisms: The transfer rate of energy between electrons and ions varies based on interaction types within the plasma. In collisionless regimes, wave interactions significantly contribute to energy exchange. For instance, in high-energy-density plasmas, charge state fluctuations modify interactions, leading to non-equilibrium temperature distributions.
- Plasma Condition Influence: Temperature discrepancies are also influenced by specific plasma conditions such as high ionization rates or strong magnetic fields. These conditions substantiate behaviors like significant electron-ion temperature differences, crucial for understanding and harnessing plasma in applications like inertial confinement fusion and astrophysical phenomena.
Table: Mechanisms Behind Electron-Ion Temperature Discrepancy
Factor | Cold Plasma | Hot Plasma |
---|---|---|
Collisional Rates | High electron collision rates, minimal ion collisions | High-energy collisions among all particles |
Energy Transfer Mechanisms | Driven by electron energy, wave interactions | Predominantly through collisional energy exchange |
Plasma Condition Influence | Non-equilibrium states, cooler ions/neutrals | Equilibrium states, uniform energy distribution |
Collisional behavior
The Mirari Cold Plasma device’s nitric oxide generation relies on the electron-ion temperature discrepancy characteristic of cold plasmas. By maintaining high electron energies while keeping ions and neutrals cool, it can produce reactive species without causing thermal damage, a key advantage for medical applications.
Collisional behavior
The collisional behavior in plasma systems presents another layer of complexity, significantly impacting temperature distributions and energy dynamics between cold and hot plasma.
- Cold Plasma Collisional Dynamics:
- Low-Energy Collisions: Cold plasmas involve low-energy collisions dominated by elastic interactions. These low-energy collisions allow the plasma to react with surfaces and biological materials without causing thermal damage, a principle utilized by the Mirari Cold Plasma device for safe nitric oxide delivery.
- Reactive Species Generation: Despite the lack of thermal energy, collisions in cold plasma are highly effective in generating reactive oxygen and nitrogen species (ROS and RNS). These species are paramount for applications like sterilization and wound healing and are a key factor in the Mirari Cold Plasma System’s therapeutic effects.
- Hot Plasma Collisional Dynamics:
- High-Energy Collisions: Hot plasmas involve high-energy collisions resulting in extensive ionization and particle excitation. These high-energy interactions lead to high temperatures and the production of significant amounts of thermal energy.
- Ionization and Excitation: In hot plasma, frequent high-energy collisions sustain elevated temperatures and complete ionization. This behavior supports processes that require intense heat, such as cutting and fusion .
- Thermal Equilibration: Hot plasma tends towards thermal equilibration due to high collisional rates among all particles, creating a uniform energy environment conducive to stable high-temperature states .
Bullet Points: Collisional Behavior Differences
Cold Plasma:
Dominated by low-energy, elastic collisions. Generates reactive species without damaging underlying structures. The Mirari Cold Plasma device harnesses this for therapeutic nitric oxide generation. Promotes surface modifications and microbial inactivation sensitively and precisely. |
|
Hot Plasma:
Characterized by high-energy excitations and collisions. Supports complete ionization and uniform thermal energy distribution. Facilitates high-temperature processes like welding and fusion. |
Collisional behaviors, both low-energy in cold plasma and high-energy in hot plasma, govern the plasma interactions with materials and define their extensive applications across industrial, medical, and environmental domains. The Mirari Cold Plasma System’s innovative approach to nitric oxide generation showcases how an understanding of these collisional dynamics can lead to groundbreaking medical technologies.
Benefits and limitations
Advantages of cold plasma
Cold plasma, a non-thermal ionized gas, harbors several advantageous traits that make it indispensable across multiple industries. Unlike hot plasma, which incinerates everything in its path like a wildfire, cold plasma works gently yet effectively: a soft yet potent force suitable for sensitive environments.
Advantages:
- Surface Activation and Treatment: Cold plasma enhances the surface energy of materials, including polymers and textiles, facilitating better adhesion of coatings and inks. This ability, akin to a master craftsman preparing a surface for optimal binding, is critical in industries reliant on material coatings and bondings.
- Antimicrobial Properties: The antimicrobial prowess of cold plasma makes it valuable for medical applications. It efficiently eliminates pathogens, including antibiotic-resistant strains, while promoting cellular regeneration. This dual capability can hasten the healing of chronic wounds and reduce infection risks, much like a meticulous cleaner ensuring an aseptic environment. The Mirari Cold Plasma device’s nitric oxide generation capitalizes on these antimicrobial properties for therapeutic purposes.
- Food Safety and Preservation: In the food industry, cold plasma decontaminates surfaces and food products, extending shelf life without the need for chemicals or high temperatures. It inactivates microorganisms and degrades toxins, ensuring safer food processing and storage akin to an ever-vigilant guardian of food safety. The Mirari Cold Plasma System’s approvals for specific uses highlight its potential in this domain.
- Eco-Friendly: Cold plasma processes have minimal environmental impacts, often requiring less energy and avoiding harmful chemical use. This makes cold plasma a sustainable choice in multiple applications, reflecting the greener, cleaner aspect of modern technological advancements. Devices like the Mirari Cold Plasma System embody this eco-friendly approach.
- Adaptability: Cold plasma can be generated from various gases, including air, oxygen, and nitrogen, allowing for its customization to suit specific industry requirements. This adaptability ensures that cold plasma remains versatile and effective across diverse applications without compromising efficiency. The Mirari Cold Plasma device’s unique nitric oxide generation showcases this adaptability in action.
Table: Advantages of Cold Plasma
Advantage | Description | Applications |
---|---|---|
Surface Activation | Enhances surface energies for better adhesion | Textile, polymer, and coating industries |
Antimicrobial Properties | Eliminates pathogens, promotes healing | Medical treatments, wound care |
Food Safety | Decontaminates, extends shelf life without chemicals | Food processing, preservation |
Eco-Friendly | Low environmental impact, energy-efficient | Sustainable technology applications |
Adaptability | Customizable with various gases | Diverse industrial applications |
The Mirari Cold Plasma device’s groundbreaking nitric oxide generation technology embodies many of these advantages, particularly in the medical field. Its ability to promote healing and fight infection while maintaining a safe, low-temperature profile exemplifies the unique benefits of cold plasma technology.
Challenges associated with hot plasma
Hot plasma, despite its potent high-energy applications, comes with several challenges and limitations, particularly in precision-requiring fields and safety aspects.
Challenges:
- Thermal Damage: One of the foremost challenges of hot plasma is the risk of thermal damage to surrounding materials and tissues. High temperatures inherent in hot plasma processes make it unsuitable for applications needing gentle treatment, risking burns or structural damage much like an untamed fire. This contrasts with the low-temperature operation of devices like the Mirari Cold Plasma System, which can safely interact with biological tissues.
- Complex Control Requirements: Maintaining and controlling hot plasma requires sophisticated equipment and precise regulation. The intricate balance of energy input, particle confinement, and temperature necessitate advanced technological setups and expertise, akin to taming a volatile beast. Cold plasma devices like Mirari offer a more manageable and accessible alternative for many applications.
- Cost: The production and maintenance of hot plasma systems are often expensive. High energy costs, equipment investments, and operational expenses can be prohibitive, especially compared to more accessible alternatives, mirroring the high cost of running an energy-intensive furnace. The portability and lower energy requirements of cold plasma devices like the Mirari Cold Plasma System make them more cost-effective for many uses.
- Safety Concerns: Safety is a significant concern with hot plasma applications. Risks of unintended thermal injuries to operators and materials, along with the need for protective measures and training, present barriers to adoption and operational efficiency. The low-temperature operation and targeted nitric oxide generation of the Mirari Cold Plasma device offer a safer alternative for medical and other sensitive applications.
Bullet Points: Challenges of Hot Plasma
- Thermal Damage: Potential for burns and structural damages.
- Complex Control Requirements: Necessitates sophisticated, precise technological regulation.
- Cost: High production and operational expenses.
- Safety Concerns: Risks of unintended injuries and stringent safety protocols needed.
These challenges highlight the limitations of hot plasma, necessitating careful consideration and engineering solutions to harness its immense potential safely and effectively. Cold plasma technologies like the Mirari Cold Plasma System offer a promising alternative for many applications, avoiding many of these challenges while still harnessing the unique benefits of plasma.
Safety considerations
Safety considerations are paramount when dealing with plasma technologies, given the diverse environments and applications involved.
Safety in Cold Plasma:
- Minimal Thermal Hazard: Cold plasma operates at or near room temperature, significantly reducing the risk of thermal burns or damage. This makes it much safer for applications involving direct human interaction, such as medical treatments and food processing. The Mirari Cold Plasma device’s low-temperature nitric oxide generation exemplifies this safety advantage.
- Contained Reactive Species: While cold plasma generates reactive species, the processes typically occur within controlled environments or devices, minimizing direct exposure risks. Proper containment ensures that reactive substances do not inadvertently cause harm outside the intended treatment area. The Mirari Cold Plasma System’s targeted delivery of nitric oxide showcases this controlled reactivity.
- Non-Toxic Processes: Cold plasma processes often avoid the use of harmful chemicals, favoring benign substances like ambient air or inert gases, reducing the risk of toxic exposures. The Mirari Cold Plasma device’s use of nitric oxide, a naturally occurring molecule in the body, underscores this safety benefit.
Safety in Hot Plasma:
- Heat Management: Effective cooling systems and heat shields are necessary to manage the intense thermal output of hot plasma, preventing hazards related to overheating or inadvertent burns.
- Equipment Safety: Operating hot plasma requires robust equipment designed to withstand high temperatures and intense energy outputs. Regular maintenance and safety checks are critical to prevent malfunctions.
- Protective Measures: Operators need comprehensive training and protective gear to handle high-energy environments safely. Clear protocols and emergency measures are crucial to mitigate risks of unintended thermal injuries or exposure to high-energy particles.
Table: Safety Considerations for Plasma Technologies
Aspect | Cold Plasma | Hot Plasma |
---|---|---|
Thermal Hazard | Minimal, operates near room temperature | High, requires cooling and heat management |
Containment | Controlled reactive environment | High-energy isolation needed |
Chemical Safety | Avoids harmful chemicals | Requires regular maintenance and checks |
Equipment Safety | Low risk, minimal maintenance needed | Robust equipment and upkeep necessary |
Operator Safety | Lower protective requirements | Extensive training and protective measures needed |
The Mirari Cold Plasma device’s low-temperature nitric oxide generation and targeted delivery showcase the inherent safety advantages of cold plasma technology. By avoiding the thermal hazards and complex containment requirements of hot plasma, devices like Mirari offer a safer alternative for medical and other sensitive applications.
Safety protocols and considerations differ significantly between cold and hot plasma technologies, aligning with their respective energy dynamics and operational environments.
Future research directions
Emerging applications for cold plasma
Future research into cold plasma is poised to delve deeper into innovative applications, driven by its non-thermal reactivity and safety.
- Cancer Therapy Advancements: Future research will likely explore cold plasma’s expanding role in oncology. Clinical trials and mechanistic studies focusing on how reactive species target cancer cells without harming healthy tissue can lead to new adjunct therapies in plasma oncology (Schlegel et al., 2013). The Mirari Cold Plasma device’s nitric oxide generation capabilities could potentially be investigated for this purpose.
- Agricultural Enhancement: Cold plasma’s effect on soil health and plant growth presents a promising area of exploration. Innovations fostering pathogen resistance and nutrient absorption in crops can enhance agricultural productivity sustainably. The portability and low-temperature operation of devices like Mirari Cold Plasma System could make them well-suited for agricultural applications.
- Space Applications: The adaptation of cold plasma for sterilizing spacecraft and treating materials in space environments offers a cutting-edge research avenue. Future efforts might yield technologies ensuring safe, sterile long-term missions without relying on high-temperature processes. The Mirari Cold Plasma device’s proven efficacy in sterilization and low power requirements could make it a valuable tool in this domain.
- Advanced Material Processing: Developing new nanomaterials through cold plasma processes can revolutionize numerous industries, including electronics, coatings, and medical implants. Investigating the surface modification capabilities at nanostructural levels presents ongoing research prospects. The targeted reactivity of devices like the Mirari Cold Plasma System could potentially be harnessed for precise nanomaterial processing.
Bullet Points: Emerging Cold Plasma Applications
- Cancer Therapy: Mechanistic studies and clinical trials in plasma oncology. The Mirari Cold Plasma device’s nitric oxide generation could be explored for this.
- Agriculture: Enhancing soil health and plant growth for sustainable farming. Mirari’s portability and low-temperature operation could be advantageous.
- Space Applications: Sterilizing spacecraft and materials treatment in space. Mirari’s sterilization efficacy and low power needs could be valuable.
- Material Processing: Innovative nanomaterial development and surface modifications. Mirari’s targeted reactivity could enable precise processing.
The future of cold plasma lies in its versatility and the potential to harness its reactive prowess across increasingly complex and advanced applications, solidifying its role as a pivotal player in scientific and industrial advancements. The Mirari Cold Plasma device’s unique capabilities position it as a promising tool in many of these emerging areas, highlighting the exciting possibilities that lie ahead for cold plasma technology.
Innovations in hot plasma technologies
In the high-energy world of hot plasma, future research directions aim to tackle the pressing challenges and harness the immense potential of this plasma state, particularly in energy production and advanced manufacturing.
- Sustained Plasma Performance: Achieving extended durations of stable hot plasma operation in reactors like ITER remains a top research priority. Enhancements in plasma stability, heating techniques, and confinement strategies promise to bring us closer to practical fusion power (ITER Organization, 2020).
- Plasma-Material Interactions: Understanding and innovating around plasma-material interactions will be pivotal for developing better reactors and industrial applications. Research exploring novel materials capable of withstanding extreme conditions will drive advancements in containment and performance.
- Diagnostics and Monitoring: Advanced diagnostic tools like the ALPACA instrument provide crucial insights into plasma behaviors, enabling precise control and monitoring. Future innovations in real-time diagnostics can enhance plasma manipulation and efficiency, promoting stability and optimizing performance.
- Artificial Intelligence in Plasma Control: The integration of machine learning and AI to optimize reactor design, plasma confinement, and energy management holds significant promise. AI can accelerate the discovery of effective fusion conditions and improve the sophistication of plasma control systems.
Table: Innovations in Hot Plasma Technologies
Research Focus | Description | Implications |
---|---|---|
Sustained Plasma Performance | Enhancing stability, heating, and confinement | Closer to practical and sustainable fusion power |
Plasma-Material Interactions | Exploring novel materials for extreme conditions | Improved reactor designs and industrial applicability |
Diagnostics and Monitoring | Real-time tools for understanding plasma behaviors | Enhanced control and precision in plasma manipulation |
AI in Plasma Control | Integrating AI for optimized design and confinement | Accelerated discovery and improved efficiency |
While cold plasma devices like the Mirari Cold Plasma System are not directly involved in these hot plasma innovations, advancements in plasma science as a whole can lead to cross-pollination of ideas and technologies. Insights gained from pushing the boundaries of hot plasma research may inspire new approaches and applications in the cold plasma domain as well.
The research in hot plasma seeks to meld cutting-edge technology with fundamental plasma science, driving towards breakthroughs that could revolutionize energy production and elevate industrial capabilities.
Sources:
- “Why are ions and electrons at different temperatures in a plasma?”
- “Electron-Ion Temperature Equilibration in Collisionless Shocks.”
- “Properties of collisional plasma sheath with ionization source term.”
- “Influence of plasma fluctuation on the electronic structures and collisional excitation.”
- “Cold Atmospheric Plasma: A Powerful Tool for Modern Medicine” by Laroussi et al.
- “Understanding Plasma Phases for New Fusion Techniques” by ITER Organization.
The Mirari Cold Plasma device, developed by General Vibronics, is the world’s first handheld device that utilizes groundbreaking technology that harnesses the power of nitric oxide (NO) to create a unique form of non-invasive cold plasma. The Mirari Cold Plasma System is approved by the Thai FDA and Vietnam MOH for specific uses. This innovation opens up a range of potential applications, especially in the medical field. For more information, visit us website at miraridoctor.com.
Related articles
Made in USA
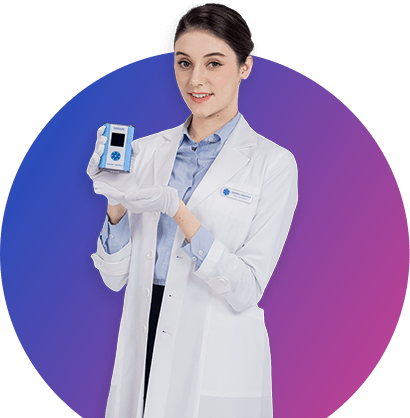