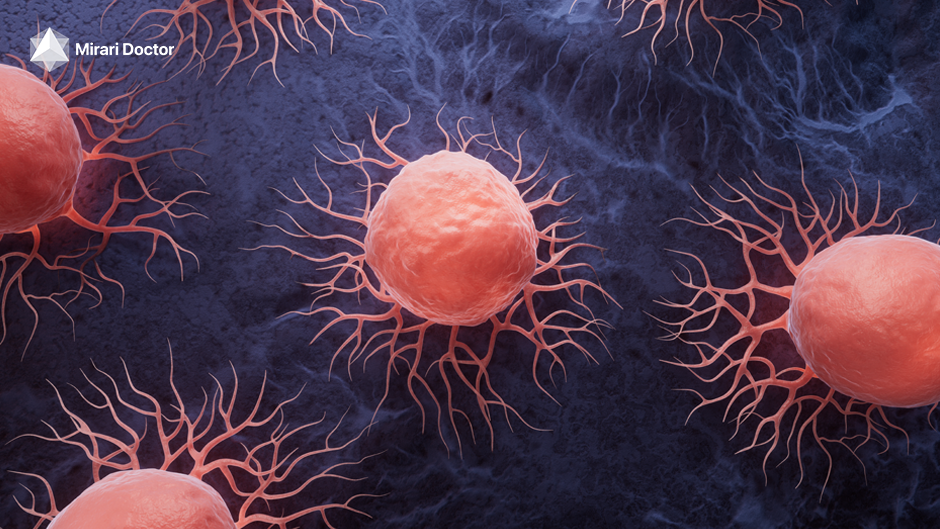
Cancer treatments have long relied on traditional methods like surgery, chemotherapy, and radiation, which, despite their efficacy, come with significant side effects and limitations. Enter cold atmospheric plasma (CAP), a novel approach garnering attention for its potential in oncological therapies. CAP operates at near room temperatures, generating reactive oxygen and nitrogen species (ROS/RNS) that selectively target cancer cells while sparing healthy tissues. Capable of activating immune responses and inducing various forms of cell death, this technology is paving the way for innovative treatment strategies.
One notable innovation in this field is the Mirari Cold Plasma device, developed by General Vibronics. This handheld device harnesses the power of nitric oxide (NO) to create a unique form of non-invasive cold plasma, opening up a range of potential medical applications. The device showcases the exciting potential of CAP technology to transform cancer treatment.
As ongoing research uncovers new insights into CAP’s mechanisms and efficacy across various cancer types, the future of cancer treatment may very well integrate this promising modality, bringing hope for more effective and less invasive cancer therapies.
Mechanisms of action of cold plasma in cancer therapy
Cold plasma’s efficacy in cancer therapy lies in its unique mechanisms of action, primarily involving reactive oxygen and nitrogen species. Think of it as an army infiltrating a fortress, expertly penetrating the defenses and dismantling its core, but leaving the surrounding village unharmed. This metaphor aptly captures the dual nature of cold plasma: its capacity to target cancer cells while preserving healthy ones. Below is a comprehensive look at these mechanisms, their chemical properties, and their biological impacts.
Chemical properties of cold atmospheric plasma
Cold atmospheric plasma is renowned for its unique chemical properties, which play a decisive role in its therapeutic potential. At the heart of plasma treatment is the creation of reactive oxygen and nitrogen species (ROS and RNS). These chemically reactive species emerge when plasma interacts with the ambient air or specific gases, leading to the formation of compounds such as hydrogen peroxide (H2O2) and nitric oxide (NO).
In conclusion, the chemical properties of cold plasma form the backbone of its anti-cancer capabilities. By understanding these properties better, researchers can optimize cold plasma treatments to increase efficacy and tailor therapies to individual patient needs.
Physical properties and their impact on tumor cells
Understanding the physical properties of cold atmospheric plasma is crucial in realizing its full potential as an anti-cancer agent. These properties include temperature, ionization, and electromagnetic fields, which together create the intricate balance needed for selective tumor targeting without harming neighboring healthy tissues.
- Temperature and Ionization:
- Cold plasma is aptly named for its operational temperature, which is near room temperature, or roughly around body temperature. This is like navigating a fine line between two extremes cold enough to avoid heat damage to tissues, yet active enough to rupture cancer cells.
- The plasma is a partially ionized gas, a mix of ions, electrons, and neutral particles. This complex mixture contributes to its reactivity and ability to interact uniquely with biological tissues. It’s almost like creating a volatile potion, lethal only when interacting with specific ingredients in this case, cancer cells.
- Generation of Reactive Species (ROS/RNS):
- At the center of cold plasma’s tumor-targeting ability is its production of reactive oxygen and nitrogen species. These species have a knack for penetrating cancer cell membranes, designed to be more permeable than their non-cancerous counterparts.
- Once inside, these reactive species act like molecular saboteurs, inducing oxidative stress and setting off a cascade of destructive biochemical reactions.
- Electromagnetic Fields:
- The presence of electromagnetic fields during the operation of cold plasma further enhances its interaction with biological tissues. These fields can influence cellular processes and signaling pathways, effectively adding another layer to its cancer-fighting arsenal.
- For instance, electromagnetic fields can help modulate the cell membrane potential, a crucial factor in the selective targeting of cancerous cells.
Mechanisms of Action on Tumor Cells
- Induction of Cell Death:
- Apoptosis: Cold plasma triggers apoptosis primarily through ROS-mediated signaling pathways, which lead to the activation of enzymes known as caspases. These caspases, in turn, jumpstart the cell’s DNA damage response mechanisms, culminating in programmed cell death.
- Necrosis and Pyroptosis: Unlike apoptosis, necrosis, and pyroptosis result in a more traumatic cell death, often causing the release of damage-associated molecular patterns (DAMPs). These DAMPs can activate the immune system, creating an anti-tumor response even after the cancer cells have died.
- Senescence: Some studies suggest that cold plasma also induces cellular senescence, a state where cancer cells are alive but no longer capable of dividing. This effectively halts tumor progression.
- Immune Modulation:
- Cold plasma’s ability to modulate the immune response is akin to rallying the body’s natural defenders. By inducing immunogenic cell death, cold plasma releases signals that recruit antigen-presenting cells and kickstart the adaptive immune response.
- This can enhance systemic anti-tumor immunity, setting off a chain reaction that helps the body identify and target remaining cancer cells.
- Enhanced Selectivity:
- Cold plasma’s selective impact on cancer cells is due to the unique properties of these cells, particularly the increased permeability of their membranes. This enhanced permeability allows ROS and RNS to infiltrate cancer cells more effectively than normal cells, creating a targeted treatment with minimal collateral damage.
The exploration of cold plasma as a cancer treatment is ongoing, with researchers striving to optimize its parameters and understand its full range of effects. As we deepen our understanding, the fusion of cold plasma’s physical and chemical properties with targeted therapies can revolutionize cancer treatment, offering a beacon of hope for more effective and less invasive solutions.
Biological responses induced by cold plasma
Cold atmospheric plasma triggers various biological responses that contribute to its effectiveness in cancer treatment. These responses, ranging from direct cellular damage to immune system modulation, illustrate the multifaceted approach of cold plasma in combating cancer.
Biological responses induced by cold plasma are diverse and multi-faceted, underscoring the technology’s potential to revolutionize cancer treatment. By combining direct cell-killing mechanisms with immune modulation and TME alterations, cold plasma offers a comprehensive strategy to target tumors effectively and with precision. As research progresses, these biological insights will guide the development of optimized treatment protocols, paving the way for cold plasma’s integration into modern oncology.
Types of cold plasma technologies
Cold plasma technologies come in various forms, each with unique mechanisms and applications. Among the most noteworthy are Dielectric Barrier Discharge (DBD), plasma jet devices, and cold plasma-activated solutions. Consider these technologies as different types of surgeons, each with their distinct tools and techniques, yet all working towards the same goal eliminating the tumor.
Dielectric barrier discharge (DBD)
Dielectric Barrier Discharge (DBD) is a prominent cold plasma technology known for its simplicity and effectiveness. It plays a crucial role in various biomedical applications, including cancer treatment. Here’s a detailed look at how DBD works and its impact on cancer therapy.
In conclusion, Dielectric Barrier Discharge represents a versatile and effective cold plasma technology for cancer treatment. Its blend of high efficacy, biocompatibility, and scalability makes it a promising tool in the fight against cancer. As research and clinical trials continue to advance, DBD’s role in oncology is expected to expand, offering new hope for patients and practitioners alike.
Plasma jet devices
Plasma jet devices represent another exciting frontier in cold plasma technology, offering precise and controllable plasma streams ideal for targeted cancer treatments. Similar to a skilled surgeon using a laser scalpel, plasma jets allow for intricate and localized interventions, enhancing the precision of cancer therapy.
- Description:
- Non-Equilibrium Atmospheric Pressure Plasma Jet (N-APPJ): Plasma jet devices, particularly the N-APPJ, generate highly directed jets of plasma that can be aimed precisely at target tissues. This capability provides an advantage in treating localized tumors without affecting surrounding healthy tissue.
- Components: These devices typically consist of electrodes and a gas feed, with common gases used including helium, argon, or nitrogen. The gas is ionized to create a plasma jet, which can be fine-tuned for specific applications.
- Mechanism:
- Plasma Generation: In plasma jet devices, the gas fed through the system is subjected to a high electric field, ionizing the gas and generating plasma. This plasma stream can be directed and focused onto the tumor site, providing high precision.
- Reactive Species Production: As with other cold plasma technologies, plasma jets produce reactive oxygen and nitrogen species (ROS/RNS). These reactive species penetrate cancer cells, creating oxidative stress and triggering cell death mechanisms such as apoptosis, necrosis, and autophagy.
- Advantages:
- Precision and Control: Plasma jets offer unparalleled precision, allowing clinicians to target tumors with minimal impact on surrounding tissues. This precision is particularly valuable in delicate areas or where tumors are located close to vital structures.
- Versatility: The adjustable nature of plasma jets makes them suitable for various applications, from superficial skin tumors to deeper lesions. This versatility enhances their utility across different cancer types and stages.
- Minimal Thermal Damage: Operating at near room temperatures, plasma jets minimize thermal damage to tissues, reducing potential side effects and enhancing patient safety.
- Applications in Cancer Treatment:
- Localized Tumor Ablation: Plasma jets excel in locally ablating tumors, offering a minimally invasive alternative to traditional surgical methods. This localized treatment reduces recovery times and minimizes complications.
- Enhanced Chemotherapy: Research has shown that plasma jet treatment can enhance the effectiveness of chemotherapy by increasing cancer cell susceptibility to chemotherapeutic agents. This synergistic approach promises improved outcomes with potentially lower drug dosages.
- Immune Modulation: Similar to other cold plasma technologies, plasma jets can stimulate immune responses, facilitating the body’s natural defenses to recognize and attack cancer cells. This immune activation adds another layer of therapeutic benefit.
Comparative Analysis of Cold Plasma Technologies
To provide a clearer perspective, let’s compare the two primary types of plasma devices DBD and Plasma Jet Devices. Below is a synthesized comparison in tabular form:
Aspect | DBD | Plasma Jet Devices |
---|---|---|
Plasma Generation | Between dielectric barriers | Directed jet of plasma |
Temperature | Near room temperature | Near room temperature |
Precision | Moderate | High |
Applicability | Large surface areas | Localized, precise targeting |
Common Gases Used | Air, other gas mixtures | Helium, Argon, Nitrogen |
Types of Cancer Treated | Various, including superficial | Localized tumors, skin cancers |
Immune Stimulation | Yes, through oxidative stress | Yes, through immune modulation |
Operational Complexity | Simple and low-cost | More complex, requires precision |
In summary, both DBD and plasma jet devices bring forward distinct advantages and applications in the realm of cancer treatment. DBD is ideal for larger surface areas and cost-effective operations, while plasma jets provide high-precision targeted therapy, making them suitable for localized and critical tumors. Together, they offer a robust toolkit for clinicians to tailor treatments to specific patient needs.
Cold plasma-activated solutions
Cold plasma-activated solutions (PAS) represent a novel application of cold plasma technology, extending its therapeutic benefits beyond direct plasma exposure. By treating liquids with cold plasma, these solutions acquire enhanced properties, primarily through the introduction of reactive oxygen and nitrogen species (RONS), making them effective against cancer cells. Think of them as elixirs, imbued with cold plasma’s potency, ready to target cancer cells systemically or topically.
- Description:
- Cold Plasma-Activated Solutions (PAS): These are liquids treated with cold plasma, resulting in solutions rich in reactive species. These reactive species confer unique therapeutic properties to the solutions, making them valuable tools in oncology.
- Commonly Used Solutions: Typical solutions activated by cold plasma include saline (saltwater), physiological buffers, or cell culture media. These liquids serve as carriers for the reactive species generated during plasma activation.
- Mechanism:
- Activation Process: The activation process involves exposing the liquid to cold plasma, allowing the generation and infusion of reactive species like hydrogen peroxide (H2O2), nitrite (NO2-), and nitrate (NO3-).
- Bioactivity: Once applied, these solutions can interact with cancer cells, inducing oxidative stress and various cell death pathways. This mechanism is similar to direct plasma treatment but offers the flexibility of liquid application.
- Advantages:
- Versatility: PAS can be applied in diverse ways, including direct application to external tumors, injection into tumor sites, or even systemically, depending on the needs of the treatment.
- Stability and Safety: Unlike direct plasma applications, PAS can be stored and used on-demand, offering a more stable and convenient option. This flexibility is crucial for clinical settings where logistical constraints may limit the immediate use of cold plasma devices.
- Applications in Cancer Treatment:
- Topical Cancer Treatments: PAS can be used topically to treat surface-level cancers such as certain skin cancers. The ability to formulate these solutions into gels or creams adds convenience and efficacy in treating localized lesions.
- Intratumoral Injections: For deeper tumors, PAS can be injected directly into the tumor site, ensuring that the reactive species are efficiently delivered to the cancer cells. This method is particularly useful for hard-to-reach tumors that are not easily accessible to direct plasma jets.
- Adjunct to Chemotherapy: PAS has shown promise in enhancing the efficacy of traditional chemotherapy drugs. By inducing oxidative stress, PAS makes cancer cells more susceptible to chemotherapeutic agents, potentially reducing the required dosage and associated side effects.
Comparative Analysis and Synergy of Cold Plasma Technologies
To encapsulate a holistic understanding, here is a comparative summary of the primary cold plasma technologies DBD, Plasma Jet Devices, and PAS:
Feature | Dielectric Barrier Discharge (DBD) | Plasma Jet Devices | Cold Plasma-Activated Solutions (PAS) |
---|---|---|---|
Primary Application | Surface tumors | Localized internal tumors | Topical, Intratumoral, Systemic |
Precision | Moderate | High | Variable, based on delivery method |
Common Gases Used | Air, various gas mixtures | Helium, Argon, Nitrogen | Aqueous solutions |
Cost and Complexity | Low | Moderate to High | Moderate |
Immune Enhancement | Yes, through oxidative stress | Yes, through immune modulation | Yes, via indirect chemical delivery |
Efficacy in Combination Therapy | Promising, with further research needed | High potential, ongoing research | Notable, often used in synergy with other therapies |
Storage and Stability | Immediate use required | Immediate use required | Stable, can be stored and used on-demand |
Thermal Impact | Minimal | Minimal | None, as it’s a liquid-based treatment |
- Enhanced Combination Therapies:
- Synergetic Potential: One of the most promising avenues for cold plasma technologies is their integration with existing cancer therapies. For example, combining PAS with chemotherapy drugs can improve drug penetration and efficacy. Plasma jet devices can be used alongside radiation therapy to enhance tumor targeting, while DBD can serve as an adjunct to surgical resection by sterilizing the surgical field and reducing the likelihood of local recurrence.
To sum up, cold plasma-activated solutions significantly broaden the scope of cold plasma applications in oncology. By offering versatile, flexible, and stable treatment options, PAS can be seamlessly integrated into various therapeutic regimes, providing targeted cancer treatment with minimal side effects. As research continues, the potential of PAS and other cold plasma technologies to revolutionize cancer treatment becomes increasingly evident.
Efficacy of cold plasma in various cancers
The efficacy of Cold Atmospheric Plasma (CAP) in cancer treatment extends across various types of cancers, with ongoing research continually unveiling new potentials. Its ability to generate reactive oxygen and nitrogen species (RONS) that induce oxidative stress and apoptosis (programmed cell death) makes CAP a versatile and promising therapeutic tool.
Devices like the Mirari Cold Plasma system are at the forefront of translating these research findings into clinical practice. By utilizing nitric oxide to create a non-invasive cold plasma, the Mirari system exemplifies how CAP technology can be made accessible and practical for medical use. Real-world applications of such devices will be crucial in validating CAP’s efficacy across different cancer types.
Below are detailed insights into the efficacy of cold plasma in skin cancer treatment, applications in gastrointestinal cancers, and effects on hematological malignancies.
Treatment of skin cancer
Skin cancer, including melanoma and non-melanoma types like basal cell carcinoma (BCC) and squamous cell carcinoma (SCC), poses a significant healthcare challenge. Traditional treatments include surgical excision, radiation, and chemotherapy, each with varying success rates and side effects. CAP offers a novel and less invasive alternative.
- Mechanisms of Action in Skin Cancer:
- Direct Cytotoxic Effects: CAP generates RONS that penetrate the outer layers of skin cancer cells, causing oxidative damage that leads to apoptosis. This mechanism is akin to a surgical scalpel made of reactive species, precisely cutting through cancer cells.
- Modulation of Tumor Microenvironment: Beyond direct cell killing, CAP affects the tumor microenvironment (TME). This alteration includes reducing angiogenesis and disrupting the extracellular matrix, making the environment less conducive to cancer growth.
- Inducing Immunogenic Cell Death: By triggering ICD, CAP not only kills cancer cells but also signals the immune system to recognize and attack them. This process can enhance local immune responses, reducing the likelihood of tumor recurrence.
- Clinical Evidence and Trials:
- In Vitro and In Vivo Studies: Various studies have demonstrated CAP’s efficacy in reducing skin cancer cell viability. In vitro studies using skin cancer cell lines show significant reductions in cell proliferation and increased rates of apoptosis after CAP treatment.
- Clinical Trials: Encouraging results from early-phase clinical trials have led to broader interest and the initiation of more extensive studies. The FDA’s approval of CAP devices for treating skin cancer marks a significant milestone, validating its efficacy and safety.
- Comparative Efficacy:
- In clinical settings, CAP has been compared to traditional treatments. For skin cancers like BCC and SCC, CAP offers a less invasive alternative with comparable efficacy to surgical excision without the complications of infection and scarring.
- Melanoma, being more aggressive, poses a greater challenge. However, CAP has shown potential in reducing tumor size and improving patient outcomes when used as a part of combination therapy with immunotherapeutic agents.
- Advantages and Future Directions:
- Non-Invasive Nature: The non-invasive application of CAP makes it particularly appealing for treating superficial skin cancers. This attribute reduces recovery times and minimizes complications.
- Potential for Combination Therapies: Research is increasingly focusing on combining CAP with other treatments. For instance, using CAP to enhance immune checkpoint inhibitor efficacy could pave the way for more effective melanoma treatments.
- Ongoing Research: Continued investigations aim to optimize CAP treatment parameters, such as exposure time and intensity, to maximize therapeutic outcomes while minimizing side effects.
Applications in gastrointestinal cancers
Gastrointestinal (GI) cancers, which include malignancies of the esophagus, stomach, and intestines, pose significant treatment challenges due to their complex etiology and often late diagnosis. Cold Atmospheric Plasma (CAP) has shown promising results in this area, especially in inducing selective tumor cell death while sparing normal tissue.
- Mechanisms of CAP in GI Cancers:
- Selective Tumor Targeting: CAP induces selective cytotoxicity in GI cancer cells by generating RONS, which disrupt the cancer cells’ oxidative balance. This disruption leads to oxidative stress, DNA damage, and eventual cell death through apoptosis or necrosis.
- Immune Modulation: CAP-treated cancer cells release damage-associated molecular patterns (DAMPs), which activate the immune system. This immune activation can lead to a more robust system-wide anti-tumor response, offering a dual-pronged attack on the cancer.
- Influence on Tumor Microenvironment: CAP can modulate the tumor microenvironment by reducing pro-tumoral factors like angiogenesis and inflammation, creating conditions less favorable for tumor progression.
- Clinical Studies and Findings:
- In Vitro and Preclinical Studies: Research in cell lines and animal models shows that CAP effectively reduces cell viability and induces cell death in various GI cancer types. These studies provide a strong foundation for clinical trials.
- Early-phase Clinical Trials: Preliminary clinical trials have demonstrated CAP’s potential in treating esophageal and colorectal cancers. These trials highlight CAP’s safety and partial effectiveness when used as a monotherapy or in combination with standard treatments.
- Comparative Efficacy:
- Against Standard Therapies: CAP’s non-invasive nature and ability to induce selective apoptosis offer advantages over traditional therapies like chemotherapy and radiation, which often come with significant side effects.
- Synergy with Chemotherapy: Studies have shown that CAP can enhance the efficacy of chemotherapeutic agents, potentially allowing for lower doses and thereby reducing side effects. This synergy provides a promising avenue for improving treatment outcomes.
- Forward-looking Perspectives:
- Combination Therapies: There is growing interest in integrating CAP with other treatment modalities, such as immunotherapy. By enhancing the tumor’s immunogenicity, CAP can make immunotherapies more effective.
- Parameter Optimization: Future research aims to refine CAP’s application in GI cancers, focusing on optimizing treatment parameters to maximize therapeutic effects and minimize harm to normal tissues.
- Regulatory Approvals and Studies: As positive results accrue, there will likely be an increase in regulatory approvals and larger-scale clinical trials, paving the way for CAP’s broader adoption in treating GI cancers.
Effects on hematological malignancies
Hematological malignancies, including leukemia, lymphoma, and multiple myeloma, present unique challenges due to their systemic nature and impact on the blood and bone marrow. CAP’s potential to induce immunogenic cell death and selectively target malignant cells offers exciting possibilities for treating these cancers.
- Mechanisms of Action in Hematological Cancers:
- Selective Cytotoxic Effects: CAP generates reactive species that induce oxidative stress and DNA damage in malignant cells. Hematological cancer cells, often characterized by high proliferative rates, are particularly susceptible to these disruptions.
- Cell Cycle Arrest and Apoptosis: CAP treatment can lead to cell cycle arrest, preventing cancer cells from proliferating. This mechanism is critical in rapidly dividing cells typical of hematological malignancies.
- Immunogenic Cell Death: By inducing ICD, CAP not only kills cancer cells but also primes the immune system to recognize and attack residual malignant cells, creating an ongoing anti-tumor response.
- Clinical Research and Evidence:
- Preclinical Studies: Studies on cell lines and animal models have shown that CAP can significantly reduce the viability of leukemia and lymphoma cells. These findings provide a compelling case for CAP’s potential in treating blood cancers.
- Initial Clinical Trials: Early-phase clinical trials are exploring CAP’s efficacy and safety in treating hematological malignancies. These trials focus on intratumoral injections of PAS and topical applications for leukemic infiltrations.
- Comparative Efficacy:
- Standard Treatments vs. CAP: Traditional treatments for hematological malignancies, such as chemotherapy and bone marrow transplants, have high toxicity and significant side effects. CAP’s targeted mechanism offers a potentially safer alternative.
- Combination Potential: Research indicates that CAP may enhance the effectiveness of existing treatments, providing a complementary approach that maximizes therapeutic outcomes while reducing adverse effects.
- Future Directions in Hematological Cancer Treatment:
- Improving Delivery Methods: Future research is likely to focus on optimizing the delivery of CAP in hematological malignancies. Innovations in this area could include using PAS for systemic treatments or combined approaches with direct plasma jets for localized treatment.
- Expanding Research: As CAP’s potential in treating blood cancers becomes more evident, larger clinical trials and expanded research efforts are expected. These efforts will be crucial in establishing standardized protocols and gaining regulatory approvals.
- Personalized Medicine Approaches: Given the variability in patient responses to treatment, CAP’s role in personalized medicine will be important. Tailoring CAP treatments to individual patients’ specific malignancy profiles could enhance efficacy and improve outcomes.
Cold plasma and immune modulation
Cold Atmospheric Plasma (CAP) has garnered significant attention for its potential to modulate immune responses, especially in the context of cancer treatment. CAP can influence the tumor microenvironment (TME) and enhance anti-tumor immunity, turning “cold” tumors into “hot” ones that are more responsive to immunotherapies.
Influence on tumor microenvironment
- Direct Impact on Tumor Cells:
- Reactive Species Production: CAP generates reactive oxygen and nitrogen species (RONS), which induce oxidative stress within tumor cells. This oxidative stress can lead to apoptosis and cell death, directly reducing tumor burden.
- Disruption of Tumor Vasculature: CAP can also disrupt the tumor vasculature, inhibiting angiogenesis. By cutting off the blood supply to the tumor, CAP stifles the nutrients and oxygen that support tumor growth.
- Modulation of Immune Responses:
- Enhanced Immune Cell Infiltration: One of the significant influences of CAP on the TME is the enhancement of immune cell infiltration. By initiating ICD, CAP releases danger signals that attract immune cells, such as T cells and natural killer (NK) cells, to the tumor site.
- Reduction of Immunosuppressive Factors: CAP can reduce the levels of immunosuppressive cytokines and cells within the TME. This reduction makes the environment less hospitable for tumor cells and more conducive to an effective immune response.
- Synergistic Effects with Immunotherapy:
- Checkpoint Inhibition: CAP can synergize with immune checkpoint inhibitors (ICIs) by making tumors more immunogenic. This synergy can potentially overcome resistance to ICIs and improve therapeutic outcomes.
- Vaccination Effect: By inducing ICD and releasing tumor antigens, CAP can create a vaccination effect, where the immune system learns to recognize and attack tumor cells more efficiently.
- Clinical Implications:
- Enhanced Immunogenicity: The ability of CAP to transform the TME from an immune-suppressive state to an immune-active one holds significant promise for improving the efficacy of existing immunotherapies.
- Potential for Combinatorial Therapies: Integrating CAP with other treatments, such as chemotherapy, radiation, and immunotherapy, could provide a comprehensive approach to tackling various cancer types.
Enhancement of anti-tumor immunity
- Immune System Activation:
- Stimulation of Dendritic Cells: CAP promotes the maturation and activation of dendritic cells (DCs), crucial for initiating adaptive immune responses. Activated DCs can present tumor antigens to T cells, fostering a robust anti-tumor response.
- Activation of T Cells: By stimulating the release of pro-inflammatory cytokines, CAP enhances T cell activation and proliferation. This activation is essential for sustaining a long-term anti-tumor immune response.
- Long-Term Immune Memory:
- Formation of Memory T Cells: CAP’s ability to induce durable immune responses suggests it can contribute to the formation of memory T cells. These cells can provide long-term surveillance against tumor recurrence, offering a sustained protective effect.
- Clinical Studies and Trials:
- Initial Findings: Early-phase clinical trials have shown that CAP can enhance the effectiveness of existing cancer therapies by modulating immune responses. These findings are paving the way for more extensive research into CAP’s role in enhancing anti-tumor immunity.
- Potential Applications: The combination of CAP with immunotherapies, such as CAR-T cell therapy and ICIs, is an active area of research. This combination could improve treatment outcomes for patients with resistant or refractory tumors.
- Advantages and Future Directions:
- Non-Invasive Enhancement: CAP’s non-invasive nature makes it an appealing option for enhancing anti-tumor immunity without the need for additional invasive procedures.
- Personalized Medicine: As understanding of CAP’s immunomodulatory effects grows, personalized approaches can be developed, tailoring CAP treatments to individual patient profiles for maximum efficacy.
Combination with immunotherapies
Combining CAP with immunotherapies holds significant potential for improving cancer treatment outcomes. This combination aims to leverage CAP’s ability to modulate the immune system, making tumors more responsive to immunotherapeutic agents.
In summary, the coupling of cold plasma with immunotherapies represents a promising avenue for cancer treatment. The potential to modulate the immune system and enhance the efficacy of existing therapies could significantly improve patient outcomes. As research progresses, these innovative combinations will likely become integral components of personalized cancer treatment strategies.
Clinical trials and FDA approvals
Cold plasma therapy’s journey from laboratory research to clinical application has reached significant milestones, including FDA approvals and successful clinical trials. These strides underscore its potential as a transformative cancer treatment.
Overview of clinical research in cold plasma therapy
- FDA Approvals and Clinical Trials:
- Initial FDA Approval: The U.S. Food and Drug Administration (FDA) granted the first clinical trial approval for cold atmospheric plasma (CAP) therapy in 2019. This approval marked a pivotal moment, integrating plasma technology into clinical oncology.
- Canady Helios Cold Plasma (CHCP): One of the pioneering devices in these trials, the CHCP system, aims to eliminate residual cancerous cells post-surgery, especially in cases where traditional methods leave behind microscopic tumors.
- First Phase I Clinical Trial:
- Trial Details: Commencing in March 2020 and concluding in April 2021, this Phase I trial featured 20 patients with stage IV or recurrent solid tumors. The primary goal was to assess the safety of CHCP treatment alongside surgical resection.
- Endpoints: Secondaryendpoints of this trial included local regional recurrence (LRR), overall survival, cancer cell death, and the efficacy of preserving adjacent healthy tissue. Results indicated promising safety profiles without adverse intraoperative incidents, and a cumulative overall survival rate of 24% was observed at the 31-month mark.
- Mechanism of Action:
- Reactive Oxygen and Nitrogen Species: CAP generates RONS, which exhibit selective cytotoxic effects on cancer cells while sparing surrounding healthy tissues. The dual nature of CAP, capable of inducing apoptosis and necrosis, further underscores its potential as a therapeutic tool.
- Broader Applications and Future Directions:
- Expanding Horizons: Beyond the initial applications in primary solid tumors like breast and lung cancers, ongoing research is exploring CAP’s use in various malignancies. The ability of CAP to interact with biological tissues and induce selective cytotoxicity highlights its potential as a versatile tool in oncological treatments.
- Future Research: Future studies aim to refine CAP applications, improve delivery methods, and develop standardized treatment protocols. This continued focus will facilitate broader acceptance and integration of CAP in clinical settings.
This overview of clinical research underscores the growing confidence in CAP’s application in oncology. The combination of safety, efficacy, and versatility positions CAP as a promising addition to the cancer treatment arsenal.
Significant findings from recent trials
Recent clinical trials have highlighted several groundbreaking findings that showcase the efficacy and potential of cold plasma therapy in treating cancer. These findings underscore CAP’s unique mechanisms of action and its role in enhancing current treatment paradigms.
- FDA-Approved Clinical Trials:
- Canady Helios™ Cold Plasma (CHCP): The Phase I clinical trial conducted by the Jerome Canady Research Institute and US Medical Innovations revealed significant tumor response in patients with stage IV or recurrent solid tumors. The CHCP device demonstrated the ability to target and eliminate residual cancer cells post-surgery effectively.
- Mechanism Validation: Studies elucidated how CHCP operates through RONS generation, causing disruptions in cancer cell viability and leading to apoptosis. The oxidation of histone mRNA and other cellular components indicated direct impacts on cancer cell processes.
- Clinical Outcomes:
- Tumor Response: Among the 20 patients treated with CHCP, significant tumor reduction was observed, showcasing CAP’s potential to complement traditional surgical methods.
- Safety and Efficacy: The trial reported a favorable safety profile, with no adverse intraoperative incidents. Furthermore, preliminary data suggested potential survival benefits, with a 24% cumulative survival rate observed at the 31-month mark.
- Presentation of Results:
- Scientific Conferences: Jerome Canady, CEO of USMI, presented these findings at the Baird Global Healthcare Conference, emphasizing CHCP’s ability to selectively target various cancers, including breast cancer. This presentation has sparked interest in further exploring personalized treatment strategies using CAP.
- Potential for Combination Therapies:
- Enhancing Standard Treatments: CAP’s ability to induce RONS and immunogenic cell death makes it an ideal candidate for combination with standard treatments like chemotherapy and radiation. This synergy can potentially improve therapeutic outcomes.
- Immunotherapy Integration: CAP’s immunomodulatory effects also suggest its potential in combination with immunotherapies, enhancing the systemic anti-tumor response and overcoming resistance to immunotherapeutic agents.
Future prospects for regulatory approvals
Looking ahead, the promising results from initial clinical trials and the growing understanding of cold plasma’s mechanisms of action set the stage for future regulatory approvals. Here are key considerations and prospects for CAP’s regulatory journey:
- Expansion of Clinical Applications:
- Phase II and III Trials: Following successful Phase I trials, there is a strong rationale for conducting Phase II and III trials to establish more extensive safety and efficacy data across diverse patient populations and tumor types. These trials will provide robust evidence required for broader regulatory approval.
- Broadening Indications: While initial trials focused on solid tumors, exploring CAP’s efficacy in hematological malignancies and other forms of cancer will be crucial. Successful trials in these areas could lead to expanded indications for CAP devices.
- Regulatory Environment:
- Favorable Precedents: The FDA’s initial approval for cold plasma trials sets a positive precedent, suggesting a receptive regulatory environment. Continued accumulation of safety and efficacy data will further strengthen CAP’s case for expanded use.
- Collaborative Studies: Collaboration with regulatory bodies and involvement in multi-center trials can accelerate the approval process. These collaborations ensure that CAP treatments meet the rigorous standards required for clinical adoption.
- Innovations in Device Technology:
- Enhancements in Device Design: Continuous innovation in the design and functionality of cold plasma devices will play a significant role in gaining regulatory approval. Improved delivery methods and enhanced safety features will address potential concerns and optimize treatment outcomes.
- Integration with Existing Technologies: Developing devices that seamlessly integrate with existing surgical and therapeutic technologies will enhance CAP’s clinical utility. Innovations in this area will facilitate faster regulatory acceptance and broader clinical adoption.
- Long-Term Research Goals:
- Understanding Combination Effects: Research focused on combining CAP with other therapies, such as immunotherapy and chemotherapy, will provide insights into synergistic effects and optimal treatment protocols. These findings will be pivotal in obtaining regulatory approvals for combination therapies.
- Standardization and Protocol Development: Establishing standardized treatment protocols and ensuring consistent application across clinical settings will be essential for regulatory approval. Long-term studies will help define these standards and validate CAP’s efficacy in diverse clinical scenarios.
In conclusion, the future prospects for regulatory approvals of cold plasma therapies are bright. The promising initial findings, combined with ongoing research and innovation, will pave the way for CAP’s broader clinical adoption. As CAP continues to prove its safety and efficacy, we can anticipate its growing role in the oncology landscape, offering new hope for cancer treatment.
Limitations and challenges
While Cold Atmospheric Plasma (CAP) holds tremendous promise as a cancer treatment, several limitations and challenges must be addressed to fully realize its clinical potential. These challenges span technical, patient-specific, and integration aspects, each presenting unique hurdles that require innovative solutions.
Technical limitations in cold plasma applications
Technical limitations pose significant challenges to the widespread adoption of CAP in clinical settings. Addressing these limitations is crucial for optimizing CAP’s efficacy and safety.
- Device Variability:
- Inconsistent Plasma Generation: Different CAP devices can produce varying levels of reactive species, leading to inconsistent treatment outcomes. This variability complicates the standardization of CAP treatments.
- Optimization of Parameters: Each CAP device requires specific operational parameters (e.g., voltage, gas type, exposure time). Optimizing these parameters for consistent and effective treatment remains a challenge.
- Standardization Issues:
- Lack of Unified Protocols: There is currently no standardized protocol for CAP treatment. Variability in treatment times, distances, and environments across different studies complicates direct comparisons and hinders the establishment of definitive efficacy.
- Treatment Delivery: Ensuring uniform delivery of CAP across different clinical settings and patient populations is essential for consistent outcomes. Developing protocols that account for patient-specific factors will be crucial.
- Tissue Penetration Limitations:
- Surface vs. Deep Tumors: While CAP is effective for surface tumors, its penetration into deeper tissues is limited. This restriction reduces its applicability for larger or more invasive tumors.
- Improving Depth of Penetration: Innovative methods to enhance CAP’s penetration, such as combining CAP with adjuvants or developing new delivery systems, are needed to extend its therapeutic reach.
- Safety Concerns:
- Thermal Damage: Although CAP operates at near room temperatures, extended exposure or close proximity to healthy tissues could cause thermal damage. Ensuring safety while maintaining efficacy is a delicate balance.
- Minimizing Side Effects: Integrating real-time monitoring and feedback mechanisms can help minimize potential side effects by adjusting treatment parameters dynamically.
Patient-specific responses and variability
Patient-specific factors significantly influence the efficacy and safety of CAP treatments. Understanding and addressing these variabilities are crucial for personalized cancer therapy.
- Genetic and Phenotypic Variability:
- Tumor Heterogeneity: Different genetic mutations and phenotypic expressions in tumors can affect their sensitivity to CAP. Tailoring CAP treatments to specific tumor profiles can enhance efficacy.
- Patient Immune Profiles: Variability in patients’ immune responses can impact the effectiveness of CAP-induced immunomodulation. Assessing individual immune profiles will aid in designing personalized treatment protocols.
- Predictability and Personalization:
- Individualized Treatment Plans: Developing personalized CAP treatment plans based on genetic, phenotypic, and immune profiles will improve predictability and outcomes. This approach requires advanced diagnostic tools and a deep understanding of CAP’s mechanisms.
- Adaptive Treatment Protocols: Implementing adaptive treatment protocols that adjust based on real-time monitoring and patient responses can improve the precision and efficacy of CAP therapy.
- Integration with Standard Cancer Treatments:
- Coordinated Care: Combining CAP with standard treatments like chemotherapy and radiotherapy requires careful coordination to maximize synergistic effects and minimize side effects.
- Balancing Efficacy and Side Effects: Standard treatments often target rapidly dividing cells indiscriminately, affecting healthy tissues. Integrating CAP with these treatments in a way that enhances therapeutic outcomes while minimizing adverse effects is a significant challenge.
Integration with standard cancer treatments
Integrating CAP into existing cancer treatment protocols presents several challenges that must be overcome to ensure its effective and safe use.
- Combination Therapy Potential:
- Enhancing Chemotherapy and Radiation: CAP has shown potential to enhance the efficacy of chemotherapy and radiation by making tumor cells more susceptible to these treatments. Establishing the optimal timing and sequence for these combinations is critical.
- Reducing Drug Resistance: CAP’s unique mechanisms can potentially overcome drug resistance in certain cancer types. Combining CAP with existing treatments could provide new avenues for patients with resistant cancers.
- Standardizing Treatment Protocols:
- Clinical Guidelines: Developing clinical guidelines for the integration of CAP with standard treatments will ensure consistent and systematic application. These guidelines should cover patient selection, treatment parameters, and safety measures.
- Training and Education: Providing comprehensive training for healthcare professionals on CAP’s use and its integration with other treatments is essential for its successful adoption. Ongoing education will keep practitioners updated with the latest research and best practices.
- Regulatory Approvals and Insurance Coverage:
- Navigating Regulatory Processes: Securing regulatory approvals for combination therapies involving CAP requires robust clinical data demonstrating safety and efficacy. Collaboration with regulatory bodies will facilitate this process.
- Insurance Reimbursement: Ensuring that CAP treatments are covered by insurance plans is crucial for their accessibility. Demonstrating the cost-effectiveness and long-term benefits of CAP will support its inclusion in insurance coverage.
In conclusion, addressing the technical limitations, patient-specific responses, and integration challenges is vital for the broad adoption of cold plasma therapy in cancer treatment. Continued research, innovation, and collaboration among researchers, clinicians, and regulatory bodies will help overcome these hurdles, paving the way for CAP’s full potential to be realized.
Future directions in cold plasma research
Cold plasma research is advancing rapidly, with emerging applications and innovations offering new possibilities in cancer treatment and beyond. The future directions in this field highlight the potential for personalized medicine, international collaborations, and novel therapeutic strategies.
Innovations like the Mirari Cold Plasma device by General Vibronics point to an exciting future where CAP technology becomes more accessible and versatile. As handheld, non-invasive devices that can harness the power of cold plasma enter the market, the potential for widespread clinical adoption grows. These devices could play a key role in realizing the vision of personalized, targeted cancer therapy using CAP.
The continuous exploration of novel applications and innovations, combined with personalized medicine approaches and international collaborations, will advance CAP’s role in cancer treatment and beyond. As research progresses, these promising avenues will contribute to the development of effective, safe, and personalized therapies that can significantly improve patient outcomes.
For those interested in learning more about cutting-edge CAP technologies like the Mirari Cold Plasma device, visit the Mirari Doctor website at miraridoctor.com. As the field evolves, staying informed about the latest innovations will be key to harnessing the full potential of cold plasma in medicine.
Novel applications and innovations
- Innovative Therapeutics in Cancer Treatment:
- Optimization of CAP Parameters: Future research will focus on refining CAP parameters, such as exposure time, distance, and atmospheric conditions, to enhance selectivity and efficacy against various cancer types. This optimization will ensure that CAP treatments are tailored to maximize therapeutic effects while minimizing side effects.
- Targeting Cancer Stem Cells: Emerging studies suggest that CAP may effectively target cancer stem cells, which are often resistant to conventional therapies. By eliminating these cells, CAP could reduce the risk of recurrence and improve long-term outcomes.
- Combination Therapies:
- Synergistic Effects with Existing Treatments: Integrating CAP with chemotherapy, radiation, and immunotherapy holds promise for enhanced treatment outcomes. Future research will explore the synergistic effects of these combinations, potentially leading to improved efficacy and reduced resistance.
- Innovative Delivery Methods: Developing new delivery methods, such as injectable hydrogels and targeted nanoparticles infused with CAP-generated reactive species, could enhance the precision and effectiveness of combination therapies.
- Wound Healing and Tissue Regeneration:
- Post-Surgical Applications: CAP’s ability to promote wound healing and tissue regeneration is crucial for post-surgical care in cancer patients. Research will focus on understanding the mechanisms by which CAP enhances cell proliferation and migration, particularly in the context of skin and soft tissue cancers.
- Chronic Wound Treatment: Beyond oncology, CAP shows potential in treating chronic wounds and ulcers, where traditional treatments often fail. Innovations in this area could lead to new therapies for patients with diabetic foot ulcers and other non-healing wounds.
- Food Safety and Preservation:
- Microbial Load Reduction: CAP’s antimicrobial properties are being explored for reducing microbial loads in food products, extending shelf life, and enhancing nutritional profiles. These applications could revolutionize food safety and preservation, with future studies aimed at regulatory approval and standardization in food processing.
- Integration with Food Packaging: Developing CAP-activated packaging materials that maintain the antimicrobial effects during storage and transportation could enhance food safety and quality.
Potential for personalized medicine
- Tailored Treatment Regimens:
- Customization Based on Tumor Profile: CAP’s specificity can be harnessed to tailor treatment regimens based on individual patient profiles, including tumor type, genetic mutations, and microenvironment characteristics. This personalized approach aims to maximize therapeutic effects while minimizing harm to healthy tissues.
- Real-Time Adjustments: Advances in diagnostic tools and real-time monitoring systems will enable adaptive treatment protocols that adjust CAP parameters based on patient responses, optimizing outcomes.
- Integration with Genomic and Immunological Data:
- Leveraging Genomic Data: Integrating CAP with genomic data can help identify patients who will benefit most from CAP therapy. Understanding the genetic mutations and molecular pathways involved in each patient’s cancer will guide personalized treatment decisions.
- Immunological Profiling: Assessing individual immune profiles will enhance the understanding of how CAP modulates the immune response. This knowledge will inform personalized immunotherapy strategies, combining CAP with immune checkpoint inhibitors, CAR-T cell therapy, and other immunomodulatory agents.
- Developing Personalized CAP Devices:
- Optimizing Device Parameters: Personalized CAP devices that adjust parameters in real-time based on patient-specific data can maximize therapeutic effects. These devices will be designed to deliver precise, controlled doses of CAP, ensuring effective and safe treatments.
- Portable and Home-Use Devices: The development of portable CAP devices for home use could enhance accessibility and convenience for patients, particularly for those requiring long-term or recurrent treatments.
International collaborations and studies
- Global Research Efforts:
- Multinational Studies: International collaborations are vital for advancing CAP research. Multinational studies and partnerships between institutions will facilitate the sharing of knowledge, resources, and technological advancements. These collaborations will accelerate the development and validation of CAP therapies.
- Standardizing Treatment Protocols: Collaborative efforts will focus on establishing standardized treatment protocols, ensuring consistent and effective application of CAP across diverse clinical settings and patient populations.
- Knowledge and Resource Sharing:
- Data Pooling: Pooling data from global studies will provide robust evidence for CAP’s efficacy and safety. This collective data will inform clinical guidelines and regulatory approvals, speeding up the translation of CAP research into clinical practice.
- Technological Innovations: Sharing technological advancements between research institutions will enhance the development of next-generation CAP devices. These innovations will improve the precision, effectiveness, and accessibility of CAP treatments.
- Regulatory Support and Approvals:
- Navigating Global Regulatory Environments: Collaborative research will support the navigation of regulatory processes in different countries, facilitating the approval of CAP therapies worldwide. Harmonizing regulatory standards will ensure the safe and effective use of CAP across various healthcare systems.
- Encouraging Regulatory Dialogue: Engaging in dialogue with regulatory bodies will foster a better understanding of CAP’s therapeutic potential and address any concerns. This proactive approach will help pave the way for future approvals and broader clinical adoption.
In conclusion, the future directions in cold plasma research are rich with potential. The continuous exploration of novel applications and innovations, combined with personalized medicine approaches and international collaborations, will advance CAP’s role in cancer treatment and beyond. As research progresses, these promising avenues will contribute to the development of effective, safe, and personalized therapies that can significantly improve patient outcomes.
In summary, the landscape of cold plasma technology in cancer treatment is rapidly evolving. Its unique mechanisms of action, ability to induce selective cytotoxicity, and potential for modulating the immune response make it a promising addition to the oncology toolkit. While significant challenges remain, continued research, innovation, and collaboration will help overcome these obstacles, paving the way for the broad clinical adoption of cold plasma therapies. Promising applications in cancer treatment, combined with potential applications in wound healing, food safety, and personalized medicine, highlight the multi-faceted potential of this technology. As the field advances, cold plasma could revolutionize several areas of medicine and healthcare, offering new hope and improved outcomes for patients worldwide.
Related articles
Made in USA
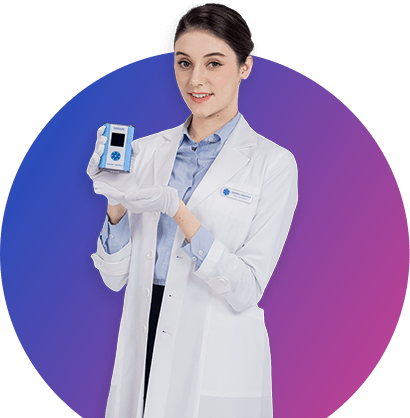