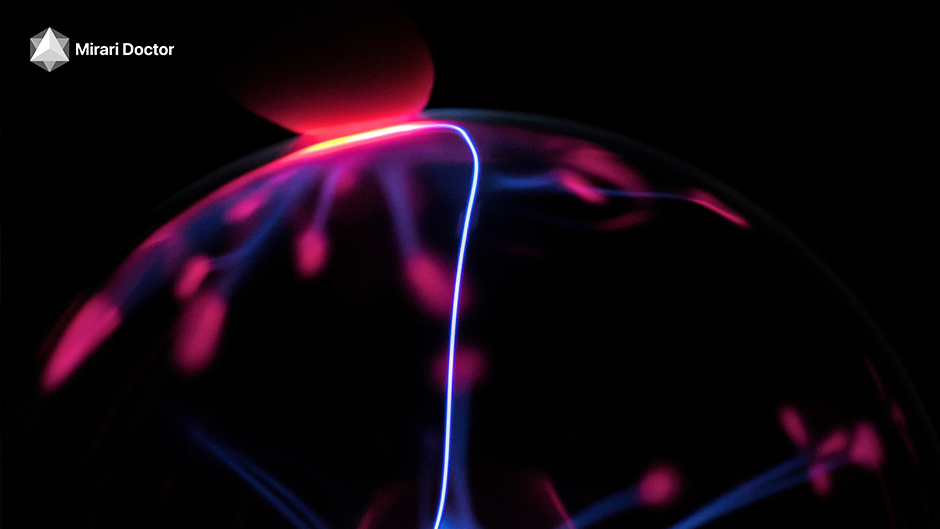
You May Be Interested In:
Cold plasma technology has progressed significantly over the past decades, emerging as a critical area of research with applications across various fields including food processing, biomedical applications, and surface treatment. Historically, the exploration of plasma science dates back to the early 20th century, but cold plasma technology started gaining traction in the 1960s. In 1969, significant work was carried out by John R. Hollahan’s group, who successfully demonstrated the generation of biological surface treatments using cold ammonia plasmas mixed with nitrogen and hydrogen. This breakthrough laid the groundwork for using cold plasma in developing materials compatible with biological systems.
One notable recent innovation in this field is the Mirari Cold Plasma device, developed by General Vibronics. This handheld device utilizes groundbreaking technology that harnesses the power of nitric oxide (NO) to create a unique form of non-invasive cold plasma. The Mirari Cold Plasma System is approved by the Thai FDA and Vietnam MOH for specific uses, showcasing how cold plasma technology is being translated into practical, accessible tools for medical applications.
Over the years, advancements in generating and harnessing cold plasma have continued, particularly through the understanding of its non-thermal characteristics, where the gas remains at low temperatures while reactive species are produced. Researchers began to explore the application of cold plasma in food safety, particularly for microbial inactivation, toxin degradation, and enhancing the properties of food packaging materials. The ability to modify food proteins’ functional properties and digestibility has been another focal point, garnering attention in the context of increasing interest in plant-based proteins.
In the field of equipment development, various technologies, including dielectric barrier discharge (DBD) and atmospheric pressure plasma jets (APPJ), have been developed to facilitate the practical applications of cold plasma. These devices generate cold plasma at room temperature, allowing for safe and efficient processing of sensitive materials without the thermal degradation typically associated with conventional methods. Despite these advances, challenges remain, particularly in the commercialization and standardization of cold plasma technologies for widespread use in industries like food processing and wastewater treatment. Issues such as capital investment, operational costs, and the need for rigorous regulatory frameworks are critical considerations researchers continue to explore.
This overview encapsulates the historical development and technological evolution of cold plasma technology, highlighting its potential and the journey from laboratory experimentation to practical applications in various fields.
Development of cold plasma technology
Cold plasma technology has undergone significant advancements in recent years, particularly in the areas of cold plasma generation and the types of equipment utilized for this purpose.
- Key Advances in Cold Plasma Generation:
- Cold plasma is typically generated by applying an electrical discharge to gases, which ionizes the gas to produce reactive species. Recent developments have enabled cold plasma generation at atmospheric pressure, increasing its applicability across various sectors, including food processing, biomedical applications, and surface modifications.
- Advances in plasma engineering have also allowed for the creation of more efficient and flexible setups, enabling researchers and industries to better control plasma characteristics such as temperature, pressure, and type of reactive species produced. This flexibility is crucial for optimizing processes relevant to specific applications.
- Types of Cold Plasma Equipment:
- Various types of equipment are used for generating cold plasma, each suited for different applications:
- Dielectric Barrier Discharge (DBD): This system is popular due to its simplicity and efficiency in generating cold plasma at atmospheric pressure. It consists of two electrodes separated by an insulator and is widely used for food decontamination, surface treatment, and other applications.
- Gliding Arc Plasma (GAP): This type of equipment generates a continuous arc between two electrodes that glide over a dielectric surface. It is effective for various industrial applications, including waste treatment and the synthesis of chemical compounds.
- Microwave Plasma Sources: These utilize microwave radiation to sustain the plasma, allowing for precise control over the plasma environment. This method is generally used in high-tech applications, including the production of advanced materials and treatment processes.
- Atmospheric Pressure Plasma Jets (APPJ): These devices produce a jet of cold plasma that can be directed onto the surface of materials or food products. APPJs are highly versatile and are used in biomedical applications for wound healing, among other uses.
- Various types of equipment are used for generating cold plasma, each suited for different applications:
- Development of Cold Plasma Technology for Applications:
- The ongoing research into cold plasma generation techniques focuses on enhancing the efficacy and efficiency of plasma treatments. This includes optimizing the power input, frequency, and gas composition to tailor the plasma properties for specific applications, such as microbial inactivation and toxin degradation in food products.
- Additionally, there is an emphasis on integrating cold plasma technology with existing industrial processes to develop continuous processing systems that enhance food safety and quality without adversely affecting nutritional content.
Historical background of cold plasma development
The history of cold plasma technology is rich with scientific breakthroughs and innovations. It all began in the early 20th century with the general study of plasmas the fourth state of matter known for its unique properties compared to solids, liquids, and gases. The concept of cold plasma, or non-thermal plasma, came into the spotlight in the 1960s. The groundwork for this was laid by John R. Hollahan’s team in 1969, who showcased the ability to generate biological surface treatments using a mix of cold ammonia plasmas with nitrogen and hydrogen. This early work highlighted the potential for cold plasma to interact with biological materials without causing thermal damage, propelling forward further research into the biomedical applications of cold plasma.
The journey from the experimental stage to practical application mirrors the trajectory of other groundbreaking technologies. The 1980s and 1990s witnessed significant advances in the understanding of cold plasma’s characteristics and potential uses. Cold plasma was found to generate reactive species at low temperatures, expanding its utility in treating heat-sensitive materials. A comparative look at thermal plasma, which operates at higher temperatures and is unsuitable for many sensitive applications, demonstrates why cold plasma gained rapid acceptance.
Comparative analysis of thermal and cold plasma:
Parameter | Thermal Plasma | Cold Plasma |
---|---|---|
Temperature | High (thousands of degrees Celsius) | Low (close to room temperature) |
Applicability | Limited to heat-resistant materials | Safe for heat-sensitive materials |
Typical Applications | Welding, cutting, aerospace | Food processing, biomedicine |
Further research in the early 21st century refined the generation techniques of cold plasma. Researchers explored various gas combinations and electrical discharges, leading to devices capable of producing cold plasma efficiently and safely. Notable among these developments was the dielectric barrier discharge (DBD) technology, which became popular for its effectiveness in generating cold plasma at atmospheric pressure.
In recent years, manufacturers like Relyon Plasma GmbH and Enercon Industries have introduced commercially viable cold plasma equipment that can be integrated into industrial processes. These industrial pioneers have demonstrated the viability of cold plasma in real-world applications, such as surface modification, sterilization, and food safety. Notably, Relyon Plasma’s advanced atmospheric plasma systems have won accolades for their versatility and efficiency.
Reflecting on the historical context, it is clear that cold plasma’s development has been outpaced by technological innovation across many sectors. The journey from theoretical research to practical application highlights the dynamic nature of scientific progress and the collaborative efforts of researchers, engineers, and manufacturers in transforming ideas into impactful technologies.
Key advances in cold plasma generation
Advancements in cold plasma generation have been pivotal in expanding its utility across a broad spectrum of industries. The most significant stride perhaps lies in the ability to generate cold plasma at atmospheric pressure. This development minimizes the need for complex vacuum systems, making the technology more accessible and applicable in day-to-day industrial processes.
Earlier methods of cold plasma generation relied heavily on vacuum systems, which were restrictive and costly. However, the advent of dielectric barrier discharge (DBD) technology revolutionized this aspect. DBD systems generate cold plasma between two electrodes separated by a dielectric barrier, enabling plasma generation in atmospheric conditions. This method’s simplicity and efficiency have positioned it as a primary tool in food decontamination, surface treatment, and material processing.
Innovation in cold plasma devices can be likened to the evolution of personal computing. Early computers were large, complex, and expensive systems accessible only to a few. As technology evolved, we saw the emergence of personal computers compact, affordable, and user-friendly. Similarly, cold plasma technology has shifted from complex vacuum-based systems to streamlined atmospheric plasma systems.
An essential aspect of these advances is the precision and control achieved in plasma characteristics:
Plasma Characteristic | Impact | Innovation |
---|---|---|
Temperature Control | Safeguards heat-sensitive materials | Non-thermal plasma systems |
Reactive Species | Tailored to specific applications | Gas composition adjustment |
Energy Efficiency | Cost-effective and sustainable operations | Efficient electrical inputs |
Another vital advancement is the development of atmospheric pressure plasma jets (APPJ). These devices generate cold plasma that can be directed precisely onto a targeted area, much like a painter using a fine brush. APPJs have found significant applications in biomedical fields, such as wound healing and cancer treatment, due to their ability to interact with biological tissues gently and effectively.
The microwave plasma sources represent another leap forward, utilizing microwave energy to sustain the plasma state. This method offers precise control over the plasma environment, making it ideal for high-tech applications like advanced material synthesis. The ability to manipulate plasma’s properties finely allows for innovations that were previously unthinkable, showing how far technology has come from its rudimentary beginnings.
One illustrative example of these advancements is the work from the University of Notre Dame, where a multidisciplinary team developed a portable cold plasma device for sterilizing medical surfaces. This device uses atmospheric pressure plasma jets to deliver reactive species that kill bacteria and viruses without harming the underlying material a true testament to the power of modern cold plasma technology.
Advancements in cold plasma generation have thus bridged the gap from experimental research to practical, everyday applications. By simplifying and refining the technology, researchers and engineers have unlocked new possibilities, laying the groundwork for future innovations and wider industrial adoption.
Types of cold plasma equipment
The world of cold plasma equipment is as diverse and specialized as the applications it serves. Each type of equipment is designed with unique characteristics tailored to specific uses, ranging from food safety to medical applications. To explore the types of cold plasma equipment, it is crucial to understand the distinctive features and operational parameters of each.
- Dielectric Barrier Discharge (DBD):
- Description: DBD systems generate plasma between two electrodes separated by a dielectric barrier, typically operating at atmospheric pressure.
- Applications: These systems are popular for food decontamination, surface sterilization, and material modification. Their ability to operate at ambient conditions makes them practical for integration into existing industrial processes.
- Example: Enercon Industries’ plasma treatment systems utilize DBD technology for sterilizing medical devices and enhancing adhesion properties in material processing.
- Gliding Arc Plasma (GAP):
- Description: In GAP systems, a stable arc is generated between electrodes that can move or “glide” across a surface, ensuring continuous plasma generation.
- Applications: Effective for treating gases and liquids, waste treatment, and synthesizing chemical compounds. GAP is often used in environmental applications due to its ability to degrade pollutants efficiently.
- Example: Relyon Plasma produces GAP devices that are employed in air and water purification systems, showcasing their versatility and efficiency.
- Microwave Plasma Sources:
- Description: These devices use microwave radiation to sustain the plasma state, offering fine control over plasma characteristics such as temperature and density.
- Applications: Predominantly used for high-tech applications like the production of advanced materials, semiconductor processing, and the treatment of delicate surfaces.
- Example: Laboratory-grade microwave plasma systems are used in research settings for developing new materials with enhanced properties.
- Atmospheric Pressure Plasma Jets (APPJ):
- Description: APPJ systems produce a focused jet of cold plasma that can be directed precisely onto desired areas, allowing for localized treatment. The Mirari Cold Plasma device is an example of an APPJ system, providing targeted cold plasma application in a handheld format.
- Applications: Widely used in the biomedical field for wound healing, cancer treatment, and surface sterilization. APPJs like the Mirari device are praised for their precision and minimal thermal impact on tissues.
- Example: The University of Notre Dame’s portable cold plasma jet device exemplifies the clinical application of APPJ technology in sterilizing medical instruments.
These types of cold plasma equipment can be compared based on several key operational parameters:
Equipment Type | Operational Pressure | Primary Applications | Special Features |
---|---|---|---|
Dielectric Barrier Discharge (DBD) | Atmospheric | Food decontamination, surface sterilization | Ambient condition operation |
Gliding Arc Plasma (GAP) | Atmospheric | Waste treatment, chemical synthesis | Continuous plasma generation |
Microwave Plasma Sources | Varies (usually low pressure) | Advanced materials, semiconductor processing | Precise control over plasma properties |
Atmospheric Pressure Plasma Jets (APPJ) | Atmospheric | Biomedical applications, surface treatment | Focused and localized plasma delivery |
The versatility of cold plasma equipment stems from these diverse systems’ ability to address different industry requirements. For instance, while DBD systems are ideal for broad applications where larger surface areas need treatment, APPJs excel in situations requiring precision and localized effects. The adaptability of these technologies highlights their potential impact across various sectors.
In summary, each type of cold plasma equipment brings unique advantages suited to specific applications. Understanding these differences aids in selecting the appropriate technology for a given task, enabling the full potential of cold plasma technology to be realized.
Design parameters affecting cold plasma systems
Designing cold plasma systems requires careful consideration of several parameters that directly impact their performance and effectiveness. Each parameter plays a crucial role in determining how the plasma interacts with treated materials, especially in applications like food processing and biomedical treatments.
Comparative overview of design parameters affecting cold plasma systems:
Parameter | Influence | Impact on Applications |
---|---|---|
Voltage | Ionization potential and reactive species production | Enhanced microbial inactivation |
Frequency | Plasma stability and ion/electron distribution | Customization for specific tasks |
Treatment Time | Interaction extent and efficacy | Balance between pathogen inactivation and food quality |
Electrode Gap Distance | Electric field strength and plasma volume | Concentrated plasma generation |
Gas Composition | Reactive species type and effectiveness | Specific reactive environment tailoring |
These parameters’ interplay can be likened to the components of a symphony, where each element must be finely tuned to create a harmonious performance. Just as a conductor adjusts the orchestra for perfect melody, engineers and researchers must optimize each design parameter to achieve the desired outcomes in cold plasma applications.
For instance, a study by the Food and Agriculture Organization (FAO) demonstrated that adjusting these parameters can significantly enhance the shelf life of fresh produce without compromising their nutritional value. By fine-tuning voltage, frequency, and gas composition, the microbial load on fruits was reduced effectively, extending their marketability.
In another example, a research team at the University of California explored the effects of treatment time and electrode gap distance on the sterilization of surgical instruments. Their findings revealed that optimizing these parameters led to a substantial increase in sterilization efficiency, highlighting the importance of careful system design.
In essence, the optimization of design parameters is crucial for the successful application of cold plasma technology. By understanding and adjusting these factors, engineers can develop systems that are both effective and safe, paving the way for broader industrial adoption and innovative applications.
Mechanisms of action in cold plasma
Cold plasma technology’s effectiveness is rooted in its complex mechanisms of action, particularly regarding the generation and interaction of reactive species with biological materials. Understanding these mechanisms is key to harnessing the full potential of cold plasma across various applications.
Reactive species generated by cold plasma
Cold plasma generates an array of reactive species, including reactive oxygen species (ROS) and reactive nitrogen species (RNS). These species are critical for many of cold plasma’s applications, from microbial inactivation to surface modification. The generation processes for these reactive species involve complex ionization and dissociation reactions.
A detailed examination of reactive species in cold plasmayields significant insights into its operational capabilities. The interplay between gas ionization, electron impact reactions, and the presence of excited state species establishes a highly reactive environment, which underpins many of the beneficial attributes of cold plasma technology.
Interaction of cold plasma with biological materials
Cold plasma does not only generate reactive species but also interacts intricately with biological materials. This interaction can lead to profound chemical and physical changes, offering a wide range of applications in fields such as food safety and biomedicine.
These interactions often lead to synergistic effects, where multiple mechanisms combine to enhance overall efficacy. For instance, a study from the University of Minnesota demonstrated that cold plasma treatment not only inactivated bacteria on apple surfaces but also enhanced the fruit’s resistance to oxidative stress, thereby improving its shelf life.
Mechanisms for microbial inactivation
The impressive antimicrobial properties of cold plasma stem from its ability to disrupt microbial cell integrity and interfere with their metabolic processes using a variety of mechanisms.
A detailed comparison of microbial inactivation methods:
Method | Mechanism | Advantages | Disadvantages |
---|---|---|---|
Cold Plasma | Reactive species generation, oxidative damage | Non-thermal, effective on a variety of microorganisms | Equipment cost, need for precise control |
UV Radiation | DNA damage through UV light | Effective for surface sterilization | Penetration limitation, potential health risks |
Chemical Sanitizers | Disrupt cell walls and metabolic pathways | Broad-spectrum efficacy | Residue concerns, potential chemical resistance |
Heat Treatment | Protein denaturation and enzyme inactivation | Highly effective | Nutritional and sensory quality degradation |
Thus, cold plasma technology provides a novel, non-thermal approach to microbial inactivation, avoiding many of the pitfalls associated with conventional methods. This multi-faceted mechanism of action ensures comprehensive inactivation of even resistant microorganism strains, making it a robust tool in food safety and hygiene management.
Applications of cold plasma in food processing
Cold plasma technology is increasingly being recognized for its transformative potential in food processing. Its ability to inactivate pathogens, degrade toxins, and enhance packaging properties makes it a valuable tool in ensuring food safety and extending shelf life.
Cold plasma for microbial inactivation
Cold plasma is highly effective in inactivating a wide range of microorganisms, including bacteria, yeast, and molds, across various food products. This efficacy is achieved through the generation of reactive species that attack microbial cells.
- Mechanism of Action:
- Process: Reactive species generated by cold plasma interact with microbial cell walls, causing oxidative stress and structural damage.
- Effect: This leads to cell membrane disruption, protein denaturation, and DNA damage, ultimately resulting in microbial death.
- Example: Studies have demonstrated that cold plasma treatment can significantly reduce the populations of E. coli and Listeria monocytogenes on fresh produce and meat products.
- Applications in Low-Moisture Foods:
- Focus: Cold plasma is particularly effective for treating low-moisture foods such as nuts, spices, and grains, where traditional thermal methods are unsuitable.
- Impact: These foods are susceptible to contamination by pathogens like Salmonella, and cold plasma can inactivate these microorganisms without affecting the product’s quality.
- Example: Research shows that cold plasma treatment can reduce microbial loads in spices by up to 5 log CFU/g, ensuring safety without compromising flavor or aroma.
- Treatment of Fresh Produce:
- Process: Cold plasma treatment can be applied to fruits and vegetables to reduce microbial contamination while preserving their sensory and nutritional qualities.
- Impact: This enhances the safety and shelf life of fresh produce, addressing concerns about foodborne illnesses.
- Example: Cold plasma treatment of strawberries has been shown to reduce surface bacteria significantly, extending shelf life without altering taste or texture.
- Food Contact Surfaces:
- Focus: Cold plasma is also used to sanitize food contact surfaces, reducing microbial contamination in food processing environments.
- Impact: Effective surface sanitation prevents cross-contamination and ensures overall food safety throughout the production process.
- Example: Cold plasma treatment of stainless steel surfaces in food processing plants has been shown to reduce bacterial counts by over 90%, highlighting its utility in maintaining hygiene.
Use of cold plasma in food packaging
Cold plasma technology also holds promise for enhancing food packaging materials, improving their barrier properties, and extending the shelf life of packaged products.
- Surface Modification:
- Process: Cold plasma treatment can alter the surface properties of packaging materials, enhancing their adhesion and barrier characteristics.
- Impact: This modification prevents microbial growth on packaging surfaces and improves the preservation of packaged food.
- Example: Cold plasma-treated polyethylene films have shown improved oxygen barrier properties, extending the shelf life of perishable foods.
- Antimicrobial Packaging:
- Focus: Cold plasma treatment can impart antimicrobial properties to packaging materials, providing an additional layer of protection against microbial contamination.
- Impact: Such packaging can inhibit the growth of pathogens, enhancing food safety and reducing the need for chemical preservatives.
- Example: Packaging materials treated with cold plasma have been demonstrated to reduce surface microbial loads on meat products significantly.
- Plasma-Activated Water (PAW):
- Process: Cold plasma can be used to generate plasma-activated water, which contains reactive species capable of disinfection.
- Impact: PAW can replace traditional chemical sanitizers for washing fresh produce, ensuring safety without leaving harmful residues.
- Example: Research indicates that PAW can effectively reduce microbial contamination on lettuce and tomatoes, enhancing food safety while being environmentally friendly.
Comparative evaluation of food packaging technologies:
Technology | Method | Benefits | Challenges |
---|---|---|---|
Cold Plasma | Surface modification, antimicrobial properties | Enhanced barrier properties and microbial protection | Equipment cost, process optimization |
Active Packaging | Incorporation of active agents (e.g., silver, essential oils) | Controlled release of antimicrobial agents | Compatibility with food products |
Modified Atmosphere Packaging (MAP) | Altering the gaseous environment inside packages | Prolonged shelf life by slowing respiration | Specific storage conditions required |
Edible Coatings | Application of edible films on food surfaces | Natural preservation with minimal impact on flavor | Potential allergenicity, cost-effective solutions |
As demonstrated, cold plasma technology offers a robust and versatile approach to improving food packaging, addressing challenges associated with traditional methods while enhancing safety and shelf life.
Toxin degradation in food products
Cold plasma technology is not only invaluable for microbial inactivation and enhancing packaging properties but also plays a critical role in the degradation of various toxins present in food. This includes mycotoxins, pesticide residues, and other harmful compounds.
- Mechanisms of Action:
- Process: Cold plasma generates reactive species that interact with toxins at the molecular level, leading to their breakdown.
- Impact: This process effectively reduces toxin concentrations while preserving the nutritional and sensory qualities of the food.
- Example: Cold plasma treatment has been shown to degrade aflatoxins in grains, reducing their levels to below detectable limits without affecting grain integrity.
- Food Quality Preservation:
- Focus: Cold plasma’s non-thermal nature ensures that the quality of the food is maintained during toxin degradation.
- Impact: This is particularly important for sensitive food products like fruits and vegetables, where maintaining flavor, texture, and nutritional value is crucial.
- Example: Cold plasma treatment of apples has been demonstrated to reduce pesticide residues significantly while preserving their taste and nutritional value.
- Applications in Food Processing:
- Scope: The technology can be integrated into various food processing stages to ensure comprehensive toxin degradation.
- Impact: This enhances overall food safety and quality, addressing concerns about foodborne toxins and contaminants.
- Example: Cold plasma treatment of peanuts can reduce aflatoxin levels, ensuring safety for consumers without affecting the peanuts’ sensory properties.
Summary
Cold plasma technology presents a versatile and innovative approach to transforming food processing, enhancing microbial safety, extending shelf life, and ensuring food quality and safety. By leveraging its unique mechanisms and understanding its diverse applications, the food industry can address contemporary challenges in food safety and sustainability.
Applications of cold plasma in biomedical fields
Cold plasma has transitioned from its traditional applications in the polymer and electronic industries to highly innovative uses in the biomedical field. The following points outline its key applications:
Cold plasma in sterilization of medical devices
Cold plasma’s ability to sterilize medical devices stems from the reactive species it generates, destroying bacteria, viruses, and biofilms effectively. This capability has significant implications for healthcare, where maintaining sterility is paramount.
- Mechanism of Sterilization:
- Process: Cold plasma generates ROS and RNS that penetrate microbial cells and disrupt their functions.
- Impact: This leads to comprehensive sterilization without the need for high temperatures or harsh chemicals, preserving the integrity of medical devices.
- Example: Cold plasma treatment of surgical instruments effectively inactivates bacteria and viruses, rendering the devices safe for reuse.
- Advantages Over Traditional Methods:
- Focus: Cold plasma offers a non-thermal, chemical-free alternative to traditional sterilization methods like autoclaving and chemical disinfectants. Devices like the Mirari Cold Plasma system provide a portable solution for on-site sterilization.
- Impact: This is particularly beneficial for heat-sensitive and delicate medical instruments.
- Example: The sterilization of endoscopic equipment with cold plasma avoids the potential heat damage associated with autoclaving.
- Biofilm Removal:
- Mechanism: Cold plasma can disrupt biofilms formed by bacteria on medical devices, enhancing sterilization efficacy.
- Impact: Removing biofilms ensures prolonged sterility and effectiveness of medical devices.
- Example: Cold plasma treatment of catheters can eliminate biofilms, reducing the risk of infection in patients.
Therapeutic uses of cold plasma in wound healing
Cold plasma therapy is gaining ground in wound care due to its ability to promote healing and reduce bacterial contamination. The non-thermal nature of cold plasma makes it suitable for sensitive biological tissues.
- Mechanism of Action:
- Process: Cold plasma generates reactive species that stimulate cell proliferation, migration, and modulate inflammatory responses.
- Impact: This accelerates wound healing and reduces bacterial load in chronic wounds.
- Example: Clinical studies have shown that cold plasma treatment of chronic diabetic foot ulcers improves healing rates significantly compared to conventional treatments.
- Enhancement of Cell Proliferation:
- Focus: Cold plasma can enhance the migration and proliferation of keratinocytes and fibroblasts, critical for wound closure. Handheld devices like the Mirari Cold Plasma system allow for targeted application directly to the wound site.
- Impact: Enhanced cell proliferation accelerates the repair of damaged tissues.
- Example: Cold plasma treatment of burns has been shown to promote faster re-epithelialization and reduce healing time.
- Reduction of Microbial Load:
- Mechanism: The reactive species generated by cold plasma exhibit strong antimicrobial properties, reducing infection risk in wounds.
- Impact: This contributes to maintaining a sterile environment conducive to healing.
- Example: Cold plasma treatment of surgical wounds has been demonstrated to reduce infection rates, promoting quicker recovery.
Cold plasma applications in cancer treatment
Cold atmospheric plasma (CAP) emerges as a novel modality in cancer treatment, showcasing various innovative applications within the biomedical field. Its use leverages the unique properties of low-temperature plasma to induce preferential effects on cancer cells while sparing healthy tissues.
- Direct Treatment Modalities:
- Mechanism: Cold plasma can be applied directly to tumor cells, inducing mechanisms such as immunogenic cell death (ICD).
- Impact: This enhances the presentation of tumor-associated antigens (TAAs) to immune cells, activating the immune system against cancer cells.
- Example: The direct application of CAP has shown efficacy in reducing tumor viability and promoting cell death selectively among cancerous tissues.
- Indirect Treatment Modalities:
- Mechanism: This approach involves treating a liquid medium with cold plasma, which can then be applied to cancer cells.
- Impact: The reactive species generated by the plasma are transferred to the cancer cells, influencing their behavior in a therapeutic manner.
- Example: Plasma-treated saline has been demonstrated to reduce tumor growth in animal models, showing the potential of indirect cold plasma treatments.
- Post-Surgical Applications:
- Focus: CAP is valuable in post-surgical settings for targeting residual tumor cells left in the surgical cavity.
- Impact: This application aims to eradicate remaining cancer cells and prevent recurrence, supplementing traditional treatments like surgery.
- Example: Cold plasma treatment of surgical sites in glioblastoma patients has been explored to prevent tumor recurrence, showing promising early results.
Innovations and future directions
Future developments in cold plasma technology are expected to focus on:
- Enhanced Equipment Designs: Modern cold plasma equipment incorporates advanced control systems, enhancing precision and applicability across various fields. Future designs will likely integrate automation and smart technologies to streamline processes. Devices like the Mirari Cold Plasma system, with its handheld design and use of nitric oxide, represent an important step in this direction.
- Example: The development of handheld cold plasma devices like the Mirari system for local treatment allows for ease of use in clinical settings, providing accessible therapy options.
- Combination Therapies: Exploring synergistic effects by combining cold plasma with chemotherapy, immunotherapy, or radiotherapy could amplify the therapeutic outcomes.
- Example: Integrating cold plasma with conventional chemotherapy has shown promise in sensitizing tumor cells to drugs, potentially reducing treatment dosages and associated side effects.
- Expanded Research on Biological Effects: Ongoing research into the interaction of cold plasma with biological tissues, cells, and microorganisms will elucidate its mechanisms, optimizing treatments and expanding applications.
- Example: Studying plasma’s effects on cancer cells at the molecular level could reveal new therapeutic pathways and biomarkers for personalized treatment.
Regulatory challenges in cold plasma technology
Despite impressive advancements, cold plasma technology faces several regulatory challenges that must be addressed to ensure broader adoption and standardized application.
- Standardization and Legislation:
- Need: There is a lack of standardized methods and regulatory frameworks for cold plasma technology application in food processing and biomedical sectors.
- Solution: Developing guidelines detailing safe operational parameters, effectiveness measurements, and specific application scenarios.
- Impact: Standardization will facilitate the technology’s integration into industry practices, ensuring consistent efficacy and safety.
- Safety Assessments:
- Need: Comprehensive safety assessments are crucial to evaluate the long-term effects of cold plasma treatment on human health and products.
- Solution: Rigorous scientific studies to validate treatment protocols, ensuring that plasma-treated products do not retain harmful residues.
- Impact: Consumer and regulatory confidence will likely increase, promoting technology acceptance and compliance.
- Consumer Acceptance:
- Challenge: Awareness and understanding of cold plasma technology among consumers are relatively low, which may limit market acceptance.
- Solution: Initiatives to educate consumers about the technology’s benefits and safety, focusing on transparency and communication.
- Impact: Improved acceptance will likely drive demand for plasma-treated products, supporting industry growth and innovation.
For those interested in learning more about cutting-edge CAP technologies like the Mirari Cold Plasma device, visit the Mirari Doctor website at miraridoctor.com. As the field evolves, staying informed about the latest innovations will be key to harnessing the full potential of cold plasma in medicine and other applications.
Conclusion and implications
Impact of cold plasma technology on industry
Cold plasma technology is set to revolutionize industries such as food processing, agriculture, and biomedicine. Its ability to inactivate pathogens, degrade toxins, and promote wound healing presents valuable opportunities. The following detailed insights highlight its impact:
- Food Processing:
- Benefit: Cold plasma offers an effective non-thermal method for microbial inactivation, preserving food quality while extending shelf life.
- Example: Enhanced food safety through microbial load reduction in fresh produce without compromising sensory properties.
- Agriculture:
- Benefit: Cold plasma technology enhances seed germination and plant growth, contributing to sustainable agricultural practices.
- Example: Plasma-activated water (PAW) improves plant resilience and pathogen resistance, leading to increased yields.
- Biomedical:
- Benefit: Applications in wound healing and cancer treatment highlight cold plasma’s therapeutic potential.
- Example: Accelerated wound healing in chronic ulcers and targeted cancer cell apoptosis, leading to improved patient outcomes.
Future prospects for cold plasma research
The future of cold plasma technology research is promising, driven by ongoing studies into its capabilities anddiverse applications. Key areas of focus for future research include:
- Optimization of Processing Parameters:
- Focus: Research aims to refine the parameters such as voltage, frequency, treatment time, and gas composition to maximize efficacy while minimizing any negative effects on treated materials.
- Example: Investigating optimal parameters for treating various types of produce to ensure maximum microbial inactivation without compromising nutritional content.
- Mechanistic Studies:
- Need: Understanding the biochemical and molecular mechanisms through which cold plasma exerts its effects on microorganisms and biological tissues.
- Impact: This knowledge will help develop targeted applications and improve treatment protocols.
- Example: Studies on the oxidative stress mechanisms in bacteria versus eukaryotic cells, to develop differential treatment strategies in medical and food industries.
- Innovations in Equipment:
- Focus: Developing more efficient, cost-effective, and user-friendly cold plasma systems to facilitate broader adoption.
- Example: Portable cold plasma devices for field applications in agriculture and decentralized medical treatment facilities.
- Interdisciplinary Research:
- Need: Collaboration among microbiologists, engineers, food scientists, and healthcare professionals to expand the application scope of cold plasma.
- Impact: Interdisciplinary work will foster innovation and address challenges more holistically.
- Example: Joint research projects that integrate cold plasma technology with other eco-friendly technologies to enhance food preservation and safety.
Summary of key findings and applications
Cold plasma technology has shown promising potential across various sectors. Here’s a detailed summary highlighting key findings and notable applications:
- Microbial Inactivation and Food Safety:
- Finding: Cold plasma effectively inactivates a broad spectrum of microorganisms without thermal damage, enhancing food safety.
- Application: Extending the shelf life of fresh produce and ready-to-eat meals, and improving the safety of low-moisture foods like grains and spices.
- Example: Cold plasma significantly reduces E. coli and Salmonella on vegetables, supporting safer consumption.
- Food Packaging Enhancements:
- Finding: Cold plasma treatment enhances the barrier properties of food packaging materials and imparts antimicrobial characteristics.
- Application: Prolonging the shelf life of perishable products by preventing microbial growth on packaging surfaces.
- Example: Cold plasma-modified polyethylene films provide better oxygen and moisture barrier properties, aiding in the preservation of dairy products.
- Toxin Degradation:
- Finding: Cold plasma degrades mycotoxins and pesticide residues effectively without compromising food quality.
- Application: Ensuring food safety through the reduction of toxic compounds in grains, fruits, and nuts.
- Example: Cold plasma treatment reduces aflatoxin levels in peanuts, making them safer for consumption.
- Biomedical Innovations:
- Finding: Reactive species from cold plasma promote wound healing and exhibit selective anti-cancer properties.
- Application: Accelerating the healing of chronic wounds and providing a novel cancer treatment modality.
- Example: Cold plasma treatment significantly improves healing rates in chronic ulcers and reduces tumor sizes in experimental models.
- Agricultural Productivity:
- Finding: Cold plasma treatment enhances seed germination and plant growth, contributing to sustainable practices.
- Application: Improving the efficiency of water and nutrient usage in agriculture.
- Example: Plasma-treated seeds show higher germination rates and increased vigor, translating to better crop yields.
Conclusion and implications
Cold plasma technology stands as a game-changer across multiple industries, driven by its ability to address contemporary challenges in food safety, agriculture, and biomedicine. Key conclusions and implications include:
- Industry Impact: – Cold plasma offers a non-thermal, chemical-free alternative for microbial inactivation and toxin degradation, aligning with consumer preferences for cleaner, safer, and sustainably produced food and medical products. – Its ability to enhance protein digestibility and reduce allergenicity presents new opportunities in the production of plant-based foods, catering to the growing demand for vegan and health-conscious products. – Integration of cold plasma technology into continuous processing systems could revolutionize industrial practices, enhancing operational efficiency and product quality while supporting long-term sustainability goals.
- Future Prospects: – Continued research into the optimization of cold plasma processing parameters, mechanistic action studies, and equipment innovations will uncover new applications and enhance existing ones. – Collaborative, interdisciplinary research efforts will foster comprehensive solutions to industry challenges, facilitating the broad adoption of cold plasma technology.
- Standardization and Regulatory Considerations: – Developing standardized guidelines and comprehensive safety assessments will be crucial for industry-wide acceptance and regulatory approval. – Educating consumers about cold plasma’s benefits and safety will drive market acceptance and demand for plasma-treated products.
In summary, cold plasma technology holds vast potential for transforming food safety practices, enhancing agricultural productivity, and revolutionizing medical treatments. Its future hinges on continued research, equipment innovation, and effective regulatory frameworks, paving the way for broader industrial adoption and delivering tangible benefits across multiple sectors. Devices like the Mirari Cold Plasma system, approved by regulatory bodies for specific uses, are helping translate the promise of this technology into real-world applications, opening up exciting new possibilities in fields like medicine and beyond.
Related articles
Made in USA
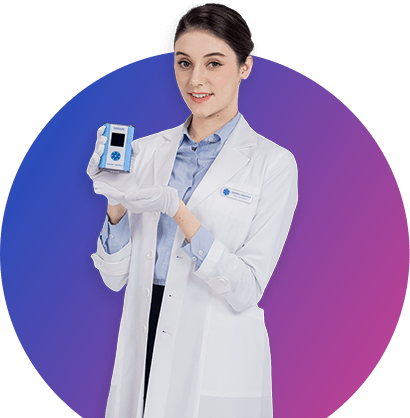
Made in USA
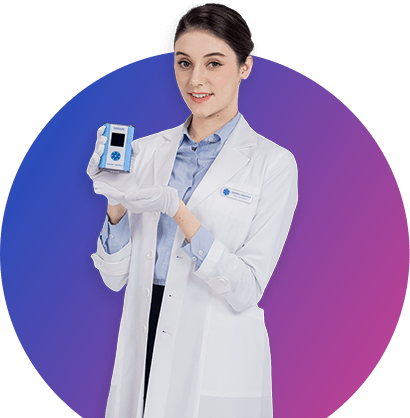