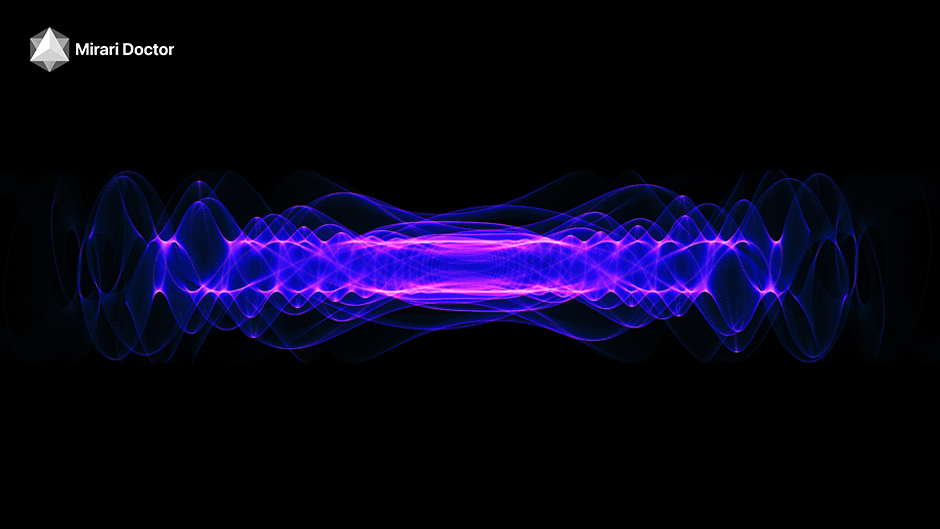
You May Be Interested In:
Cold plasma, or non-thermal plasma, has rapidly advanced from scientific laboratories to a variety of practical applications across numerous industries.[1] Characterized by its lower temperatures and unique chemical properties, cold plasma stands out not just as a technological marvel but also as an essential tool for the future.[2] From food safety enhancements to breakthrough treatments in medicine and agriculture, cold plasma’s versatility is akin to a Swiss army knife, offering multiple functionalities seamlessly.[3] As we embark on this comprehensive exploration of cold plasma technology, we aim to uncover its principles, operational mechanisms, and widespread applications. This article will delve into the fundamentals of cold plasma, examine its various devices, and highlight its transformative impact across food, medical, and industrial sectors. Furthermore, we will explore potential future directions and the challenges that lie ahead, rounding out our deep dive into this captivating subject.[4]
One notable innovation in the field of cold plasma technology is the Mirari Cold Plasma device, developed by General Vibronics.[5] This handheld device utilizes groundbreaking technology that harnesses the power of nitric oxide (NO) to create a unique form of non-invasive cold plasma. The Mirari Cold Plasma device opens up a range of potential applications, especially in the medical field, offering a portable and user-friendly solution for healthcare professionals and patients alike.[6] While this article will provide a comprehensive overview of cold plasma technology and its diverse applications, we will also touch upon how innovations like the Mirari Cold Plasma device are pushing the boundaries of what’s possible with this remarkable technology.
Fundamentals of Cold Plasma Technology
Understanding the fundamentals of cold plasma technology is akin to discovering the building blocks of an enigma. Cold plasma, often referred to as a ‘fourth state of matter,’ exists in a state where energy is absorbed mainly by electrons rather than by molecules, making it vastly different from the hot plasmas found in stars or fire.[7] Comparing cold plasma to a gentle breeze that holds the power of a hurricane captures its essence and potential when harnessed correctly. Cold plasma operates at near-room temperature, and its non-thermal nature makes it suitable for applications that traditional high-temperature plasmas would damage.[8] With a palette of reactive substances like ions, free radicals, and UV photons, cold plasma can be meticulously controlled and tailored, allowing for precise application across fields ranging from delicate food items to sensitive medical treatments.[9]
Definition and Characteristics of Cold Plasma
Cold plasma is defined as a partially ionized gas consisting of ions, electrons, and neutral particles, which exists under low-temperature conditions. Unlike thermal plasma, where higher temperatures are necessary for ionization, cold plasma can be generated at atmospheric pressure and low temperatures, typically not exceeding 40 °C.[10] The widespread application of cold plasma stems from its ability to ionize gases using electromagnetic fields, such as radiofrequency (RF) or microwave energy, creating a versatile technology that operates under low-energy conditions. This capability allows for the treatment of heat-sensitive materials without causing damage.[11]
When we think of cold plasma’s characteristics, several key features emerge:
![]() |
Non-thermal Nature:
The defining characteristic of cold plasma is its minimal thermal load, making it ideal for applications where heat could potentially cause damage, such as in the food and medical industries.[12] |
![]() |
Reactivity:
Cold plasma is a powerhouse of reactive species, including ions, electrons, radicals, and ultraviolet (UV) light, which drive its efficacy in disinfection, surface activation, and chemical modifications.[4] |
![]() |
Control over Parameters:
The versatility of cold plasma lies in its ability to finely control various operational parameters such as voltage, frequency, gas composition, and treatment duration. This flexibility enables customized applications tailored to specific needs.[13] |
![]() |
Versatility of Applications:
Beyond basic applications, cold plasma has been a game-changer in numerous fields: food safety and preservation, medical sterilization and treatment, and even agricultural enhancements.[14] |
The fundamental mechanisms governing cold plasma’s interaction with materials derive from the specific reactive species produced and how these interact with treated surfaces or food items. Energetic electrons in cold plasma play a pivotal role in dissociating gas molecules, creating reactive radicals that initiate complex chemical reactions.[15] For instance, in food decontamination, these reactive species disrupt the cellular structure of pathogens, effectively neutralizing them while retaining the food’s quality.[16]
Take, for example, its application in the food industry. Studies have documented over 5-logarithmic reductions in pathogens like Salmonella and Escherichia coli O157:H7 within a matter of minutes, eliminating these harmful microorganisms without altering the food’s physical or nutritional properties.[17] This precision and control offer a glimpse into why cold plasma is considered a cornerstone technology for future innovations.
In summary, cold plasma technology represents an extraordinary convergence of physics, chemistry, and engineering, providing innovative solutions and immense potential across diverse industries.[18]
Mechanisms of Cold Plasma Generation
Harnessing the power of cold plasma begins with understanding its generation mechanisms, which can be likened to the ignition process of a finely-tuned engine. Electric discharge methods are the heart of cold plasma generation, making use of gases such as air, argon, helium, and oxygen subjected to varying electric fields to create plasma.[19]
![]() |
Electrical Discharge Methods:
Dielectric Barrier Discharge (DBD): This technique involves placing a dielectric material between two electrodes and applying a high voltage across them. When the electric field strength reaches a critical point, it ionizes the gas, creating micro discharges. DBD is prized for its low energy consumption and compatibility with atmospheric pressure, facilitating its extensive use in surface sterilization and food processing.[20] Glow Discharge: Unlike DBD, glow discharge involves applying voltage across electrodes in a low-pressure environment, ionizing the gas to form plasma. This method is appreciated for its homogeneous plasma generation at lower temperatures, finding applications in coatings and material processing.[21] |
![]() |
Atmospheric Pressure Plasma Jet (APPJ):
This technique produces a high-velocity jet of plasma via a high-voltage electric field applied to a gas, allowing the plasma to be expelled into the surrounding atmospheric conditions. APPJ’s precision makes it particularly effective in biomedical applications, such as wound treatment, where controlled and localized plasma delivery is paramount.[23] |
![]() |
Plasma Arc Technology:
This method involves creating a continuous arc between two electrodes within a gas, generating high-temperature plasma that can be rapidly cooled to produce cold plasma. While primarily used in industrial applications due to its high energy output, plasma arc technology showcases the robust adaptability of cold plasma generation methods.[7] |
Types of Cold Plasma Devices
Cold plasma devices cater to a multitude of applications, each uniquely designed to leverage specific plasma generation methods. Below is a comparison of various cold plasma devices, underscoring their distinctions and advantages[12]:
Device Type | Typical Uses | Remarks |
---|---|---|
Dielectric Barrier Discharge (DBD) Devices | Food processing, Surface sterilization | Effective in microbial cell disruption with minimal impact on food quality[24] |
Plasma Jet Devices | Wound healing, Cancer treatment | Precise and localized treatment with reduced thermal impact on surrounding tissues[18] |
Low-Pressure Plasma Reactors | Semiconductor fabrication, Surface treatment of materials | High uniformity plasma generation ideal for large area treatments[25] |
Plasma-Based Coating Systems | Thin film applications textile and automotive industries | Enhances surface properties, providing durable and functional coatings[26] |
The Mirari Cold Plasma device is a unique handheld device that utilizes nitric oxide (NO) to generate non-invasive cold plasma. Its portability and ease of use make it particularly well-suited for medical applications, allowing healthcare professionals to deliver targeted cold plasma treatments in various settings. The device’s innovative use of nitric oxide sets it apart from other cold plasma devices, offering a new approach to harnessing the benefits of this technology.[5]
To illustrate further, Dielectric Barrier Discharge (DBD) devices have emerged as reliable tools in food processing, offering the ability to disrupt microbial cells effectively. The low-temperature plasma generated in these devices ensures that the food’s quality remains uncompromised, a significant advantage over traditional thermal methods that might degrade food components.[9]
Plasma Jet Devices, on the other hand, excel in medical applications. By delivering a continuous stream of cold plasma, these devices allow for precise targeting required in complex treatments like wound healing and cancer therapy. Plasma jets provide the added benefit of minimal heat, reducing the risk of damage to adjacent tissues, which is crucial for patient recovery and treatment outcomes.[27]
Low-Pressure Plasma Reactors are invaluable in the semiconductor industry, providing a controlled vacuum environment that facilitates uniform plasma treatment over large surfaces. This uniformity is crucial for applications such as thin-film coatings, where consistency directly impacts the quality and functionality of the final product.[28]
Lastly, Plasma-Based Coating Systems leverage cold plasma to create functional, thin-layer coatings that significantly enhance the surface properties of textiles and automotive components. These systems improve durability, resistance, and even aesthetic appeal, illustrating cold plasma’s broad industrial applicability.[29]
In summary, the variety and specificity of cold plasma devices epitomize the technology’s adaptability, offering tailored solutions for diverse applications across food processing, medical treatments, and industrial manufacturing. Innovations like the Mirari Cold Plasma device are expanding the possibilities even further, providing healthcare professionals with a powerful new tool to deliver the benefits of cold plasma technology.
Applications of Cold Plasma in The Food Industry
Cold plasma technology is emerging as a versatile solution in the food industry, particularly in areas such as food decontamination, preservation, and packaging. The applications of cold plasma in the food sector are multifaceted, addressing both safety and quality concerns while offering environmentally friendly solutions.[24]
![]() |
Food Decontamination:
Cold plasma is utilized to eliminate pathogens and spoilage organisms from various food products, including fruits, vegetables, meats, and poultry. This non-thermal technology can achieve significant reductions in microbial loads, often reaching over 5-logarithmic reductions for pathogens like Salmonella and Escherichia coli O157:H7 in a matter of seconds to minutes. It operates by generating reactive gases that disrupt microbial cell structures without affecting the food’s integrity or quality.[17] |
![]() |
Enzyme Inactivation:
Cold plasma processing can effectively inactivate enzymes responsible for spoilage and degradation in food products. This contributes to longer shelf lives and maintains food quality during storage. The specificity of the plasma treatment allows for targeted actions on enzymes while minimizing changes to desired qualities in food.[30] |
![]() |
Toxin Degradation:
Toxin degradation in food processing is another critical area where cold plasma proves beneficial. By degrading mycotoxins and other harmful substances in food products, cold plasma ensures safer consumption, particularly important for crops vulnerable to such contaminants.[31] |
![]() |
Packaging Modifications:
Cold plasma technology can be applied to modify packaging materials to enhance their barrier properties or add antimicrobial surfaces. This adaptability can lead to longer shelf life for packaged foods while ensuring safety against microbial contamination.[9] |
![]() |
Surface Sterilization:
Cold plasma can sterilize food surfaces and equipment in processing plants. This helps in maintaining hygiene standards and reducing cross-contamination during food processing.[14] |
![]() |
Quality Enhancement:
Cold plasma processing positively influences food quality attributes such as color, texture, and nutrients. By manipulating the parameters of cold plasma treatment, food manufacturers can achieve desired quality traits while ensuring safety.[32] |
Cold plasma’s array of reactive species, including ions and UV light, interact effectively with microorganisms and contaminants while maintaining the food products’ thermal stability. This technological marvel is testament to its potential to revolutionize food safety, extend shelf life, and enhance food quality.[16]
Food Decontamination Methods
Cold plasma technology has proven to be a powerful tool in food decontamination, ensuring food safety without compromising quality. It provides an alternative to traditional thermal methods, offering several advantages such as minimal nutrient loss and maintaining the sensory properties of foods.[24]
![]() |
Mechanisms of Decontamination:
The primary mechanism of cold plasma’s decontamination power lies in its production of reactive species. These reactive oxygen and nitrogen species (RONS) are highly effective at disrupting microbial cell walls and membranes, leading to the inactivation of pathogens.[4] The interaction with microbial DNA and proteins further contributes to microbial cell death, ensuring comprehensive microbial inactivation.[33] The non-thermal nature of cold plasma means that it doesn’t raise the temperature of the food, avoiding thermal degradation of heat-sensitive components.[3] |
![]() |
Comparison with Traditional Methods:
Heat Treatment: Traditional heat treatment methods can degrade the quality of food, particularly affecting vitamins, proteins, and the overall sensory properties. In contrast, cold plasma offers a non-destructive alternative.[34] Chemical Treatments: While chemical treatments such as chlorine washing can be effective, they pose food safety concerns related to chemical residues. Cold plasma, on the other hand, does not leave such residues, offering a cleaner method of decontamination.[16] High-Pressure Processing (HPP): Although HPP is effective, it’s an expensive process and still may alter textural properties of food. Cold plasma, in comparison, is more cost-effective and better at preserving food texture.[35] |
![]() |
Applications and Effectiveness:
Fruits and Vegetables: Studies demonstrated cold plasma’s effectiveness in reducing microbial loads on fresh produce without affecting their quality. For example, researchers have shown that cold plasma treatments on strawberries and lettuce can achieve significant reductions in E. coli and Salmonella.[17] Meat and Poultry: Cold plasma treatment effectively decontaminates meat and poultry surfaces from pathogens like Listeria and Campylobacter, enhancing food safety while retaining the meat’s freshness and nutritional value.[36] Dairy Products: Its application in dairy processing can significantly reduce spoilage organisms without altering nutritional and sensory properties, which are often delicate and sensitive to heat.[37] |
![]() |
Optimization of Parameters:
Optimizing parameters such as gas type, flow rate, exposure time, and power settings is crucial for maximizing the efficacy of cold plasma treatments. For different food matrices, these parameters need adjustment to ensure effective decontamination without compromising quality.[14] |
![]() |
Implementation Challenges:
While promising, cold plasma technology faces implementation challenges such as cost of equipment, scalability for large-scale operations, and regulatory approvals. Addressing these challenges requires continued research and development, as well as collaboration between industry stakeholders.[9] |
Enhancing Food Quality With Cold Plasma
Cold plasma technology not only ensures safety but can also enhance various quality aspects of food, making it a vital tool for modern food processing.[38]
![]() |
Microbial Inactivation and Shelf Life Extension:
Cold plasma treatment significantly reduces microbial load, which is critical for extending the shelf life of food products. This is especially beneficial for fresh produce, meats, and dairy products, where microbial spoilage limits shelf life.[17] |
![]() |
Preservation of Sensory and Nutritional Qualities:
Unlike thermal processing methods, cold plasma maintains the sensory attributes such as color, texture, and flavor of food. For instance, cold plasma treatment of tomatoes has preserved their sensory properties while eliminating surface bacteria.[32] Nutritional components such as vitamins, antioxidants, and proteins are preserved during cold plasma treatment, as there is minimal heat generation. This makes it an advantageous method for maintaining the nutritional integrity of fruits and vegetables.[39] |
![]() |
Protein Modification and Digestibility:
Cold plasma treatment can modify the structure of food proteins, enhancing their digestibility. For example, studies have found that plasma treatment of whey protein improved its digestibility by breaking down protein structures to facilitate enzymatic action.[40] |
![]() |
Enzyme Inactivation:
Cold plasma effectively inactivates enzymes that cause spoilage and degradation in food products. This contributes to longer shelf life and preserves food quality during storage.[30] For example, the inactivation of polyphenol oxidase (PPO) and peroxidase (POD) enzymes in fruits and vegetables helps in preventing browning and maintaining the visual appeal of the products.[34] |
![]() |
Optimization of Treatment Parameters:
The effectiveness of cold plasma in enhancing food quality depends on optimizing various treatment parameters such as gas composition, exposure time, and power settings. Tailoring these parameters for specific food products ensures the desired quality improvements while maintaining safety standards.[14] |
![]() |
Applications in Different Food Products:
Fruits and Vegetables: Cold plasma treatments have been effective in enhancing the quality of strawberries, tomatoes, and lettuce by reducing microbial load and preserving sensory properties.[35] Meat and Poultry: Cold plasma helps in retaining the freshness, flavor, and nutritional value of meat and poultry products by reducing spoilage organisms.[36] Dairy Products: The application of cold plasma in dairy processing helps in maintaining the quality and extending the shelf life of products like milk, cheese, and yogurt.[37] |
While cold plasma technology has demonstrated its effectiveness in enhancing food quality across various products, it’s important to note that different food matrices may require specific optimization of treatment parameters. For instance, the Mirari Cold Plasma device, with its unique nitric oxide-based cold plasma generation, may offer distinct advantages for certain food applications.[5] As research continues to expand the possibilities of cold plasma technology, food manufacturers can explore how devices like Mirari can be integrated into their processes to achieve optimal results in terms of food safety, quality, and shelf life extension.
Toxin Degradation in Food Processing
The degradation of toxins in food is a crucial application of cold plasma technology, ensuring the safety and quality of food products.[24]
- Mechanisms of Toxin Degradation:
- Cold plasma generates reactive species such as reactive oxygen and nitrogen species (RONS), which can breakdown mycotoxins and other harmful substances in food. These species interact with the chemical bonds of toxins, leading to their degradation.[4]
- Effectiveness against Mycotoxins:
- Mycotoxins such as aflatoxins and ochratoxin A are produced by fungi and can contaminate crops like grains and nuts. Cold plasma treatment has proven effective in degrading these mycotoxins, ensuring safer consumption of such food products.[41]
- Studies have shown significant reductions in aflatoxin levels in plasma-treated peanuts and maize, highlighting the potential of cold plasma technology in managing mycotoxin contamination in various food crops.[31]
- Environmental Safety:
- Cold plasma offers an environmentally friendly method for toxin degradation as it does not require harsh chemicals and produces minimal waste. This aligns with sustainable food processing practices making cold plasma an attractive option for food safety interventions.[16]
- Application Versatility:
- Cold plasma technology is adaptable for a variety of food types, including low-moisture foods, where traditional methods may be less effective. For instance, plasma treatment has shown efficacy in degrading toxins in dried fruits and nuts, which are particularly susceptible to mycotoxin contamination.[42]
- The ability of cold plasma to penetrate and treat food surfaces makes it suitable for comprehensive toxin remediation.[43]
- Combination with Other Technologies:
- Cold plasma can be combined with other food processing technologies to enhance its effectiveness. For example, integrating plasma treatment with ultrasonication or pulsed light can provide a synergistic effect, improving the degradation of toxins and ensuring higher food safety standards.[44]
- Optimization of Treatment Parameters:
- Just like in other applications, optimizing the parameters such as gas type, flow rate, exposure time, and voltage is critical for maximizing the efficacy of cold plasma treatments in toxin degradation. Different food matrices require specific adjustments to ensure effective toxin degradation without compromising food quality.[14]
Innovations in cold plasma devices, such as the Mirari Cold Plasma device, are expanding the possibilities for targeted toxin degradation in food processing. The device’s unique nitric oxide-based cold plasma generation may offer distinct advantages in breaking down specific toxins while maintaining food quality.[5] As research progresses, the integration of advanced cold plasma devices into food processing protocols can significantly enhance food safety by effectively mitigating the risks associated with mycotoxins and other harmful substances. This not only benefits consumers but also supports the food industry’s efforts to provide safer, high-quality products to the market.
Surface Modifications of Packaging Materials
Cold plasma technology has significant applications in the food industry, particularly in the surface modification of packaging materials. This process involves altering the surface properties of materials to enhance functionality and improve food preservation and safety.[9]
- Enhancement of Barrier Properties:
- Cold plasma treatment modifies the surface properties of packaging materials, enhancing attributes like adhesion, wettability, and barrier properties. These modifications can lead to the development of packaging systems with improved resistance to gases and moisture, thereby extending the shelf life of packaged foods.[45]
- For example, plasma-treated polyester films display better oxygen barrier properties, reducing the oxidation rate of packaged food items.[46]
- Antimicrobial Properties:
- Cold plasma can impart antimicrobial properties to packaging materials by embedding reactive species on the surface. These reactive species effectively inhibit the growth of microorganisms on the packaging, contributing to the safety of the food product.[47]
- Studies have shown that plasma-treated polyethylene films exhibited significant reductions in microbial growth compared to untreated films, underscoring the potential of plasma-modified packaging in improving food safety.[48]
- Resistant Properties Against Biofilm Formation:
- Biofilm formation on the surfaces of food packaging can pose significant risks to food safety. Cold plasma treatments can help inhibit biofilm formation by effectively functionalizing surfaces to resist microbial adhesion and growth.[49]
- Plasma-treated packaging materials have shown resistance to biofilm formation by common foodborne pathogens, ensuring better hygiene and product quality during storage and transport.[50]
- Innovative Functional Polymers and Coatings:
- The versatility of cold plasma allows for the creation of novel functional polymers and coatings tailored for food packaging applications. These coatings deliver enhanced properties such as improved strength, barrier performance, and antimicrobial activity.[51]
- For instance, plasma-deposited nanocoatings can provide excellent gas barrier properties while maintaining the transparency and mechanical integrity of the packaging material.[52]
- Sustainable and Eco-Friendly Approach:
- Cold plasma surface modification offers a sustainable and eco-friendly alternative to traditional chemical treatments. Plasma processes reduce the reliance on harmful chemicals, minimizing the environmental impact of packaging production.[38]
- By enhancing the properties of biodegradable and recyclable packaging materials, cold plasma technology supports the food industry’s efforts to adopt more sustainable practices.[53]
- Optimization of Treatment Parameters:
- The effectiveness of cold plasma surface modification depends on optimizing various treatment parameters, including plasma gas composition, power input, exposure time, and substrate properties. Fine-tuning these parameters ensures the desired surface properties are achieved while maintaining the integrity of the packaging material.[54]
The Mirari Cold Plasma device, with its innovative nitric oxide-based cold plasma generation, may offer unique advantages in surface modification of food packaging materials. The device’s ability to generate highly reactive species could potentially enable the creation of advanced functional coatings that enhance barrier properties, antimicrobial activity, and biofilm resistance.[5] As research continues to explore the capabilities of devices like Mirari, the food packaging industry can benefit from new opportunities to develop high-performance, sustainable packaging solutions that extend product shelf life and ensure food safety.
Cold Plasma in Medical Applications
Cold plasma technology is making groundbreaking strides in the medical field, providing innovative solutions to several critical healthcare challenges. Its non-thermal properties and ability to generate reactive species make it particularly suited for sensitive medical applications, such as wound healing, cancer treatment, and sterilization of medical equipment.[1]
- Integration in Clinical Practices:
- Cold plasma has been integrated into various clinical practices, demonstrating its versatility and effectiveness. From promoting wound healing to targeting cancer cells, cold plasma technologies provide non-invasive and efficient solutions that enhance patient outcomes.[55]
- FDA-approved Trials:
- The FDA has approved numerous trials exploring the potential of cold plasma in medical applications. Notably, trials in cancer treatment have shown promising results, positioning cold plasma as a potential breakthrough therapy.[56]
- Biomedical Engineering:
- Advances in biomedical engineering have facilitated the development of specialized cold plasma devices tailored for medical applications, ensuring precise and safe utilization of this technology.[57]
Cold Plasma for Wound Healing
Cold plasma has emerged as a revolutionary treatment for wound healing, showcasing impressive results in both chronic and acute wounds.[58]
![]() |
Generation of Reactive Species:
Cold plasma generates reactive species such as reactive oxygen and nitrogen species (RONS) that play a crucial role in accelerating wound healing processes. These reactive species stimulate cellular activities, including increased fibroblast proliferation, angiogenesis, and collagen synthesis.[59] |
![]() |
Reduction of Infection and Inflammation:
Cold plasma exhibits potent antimicrobial properties that reduce infection and inflammation in wounds. Studies have shown that cold plasma treatment can eliminate a broad spectrum of pathogens, including antibiotic-resistant strains like MRSA, from chronic wound surfaces.[60] The reduction of infection leads to a decrease in inflammation, creating an optimal environment for wound healing and tissue regeneration.[61] |
![]() |
Enhanced Tissue Regeneration:
The tissue-stimulating effects of cold plasma promote faster wound closure and tissue regeneration. For example, studies have demonstrated improved healing rates in diabetic ulcers and pressure sores following cold plasma treatment.[62] |
![]() |
Minimization of Pain and Discomfort:
The non-thermal nature of cold plasma minimizes pain and discomfort during treatment, making it suitable for patients with sensitive or painful wounds.[64] Clinical applications of cold plasma in wound care have shown patient reports of reduced pain, contributing to improved patient compliance.[65] |
The Mirari Cold Plasma device, with its innovative nitric oxide-based cold plasma generation, offers a promising solution for wound healing applications. Nitric oxide is known for its role in promoting vasodilation, enhancing blood flow, and stimulating the production of growth factors crucial for tissue repair.[66] By harnessing the power of nitric oxide, the Mirari device may provide a targeted and effective approach to accelerate wound healing and improve patient outcomes.[5]
Applications in Cancer Treatment
Cold plasma is showing promise as a novel approach in cancer treatment, offering targeted and effective therapy with minimal side effects.[67]
- Selective Targeting of Cancer Cells:
- One of the critical advantages of cold plasma therapy is its ability to selectively target cancer cells while sparing healthy tissues. The reactive species generated by cold plasma induce apoptosis (programmed cell death) in cancer cells without harming surrounding healthy cells.[68]
- Clinical studies have demonstrated the efficacy of cold plasma in reducing tumor size and promoting tumor cell death in various cancer types, including melanoma, breast cancer, and lung cancer.[56]
- Induction of Apoptosis:
- The reactive species produced by cold plasma disrupt critical cellular processes in cancer cells, leading to oxidative stress and DNA damage. This disruption triggers apoptosis, effectively inhibiting cancer cell proliferation and tumor growth.[27]
- The specificity of cold plasma’s action on cancer cells enhances its potential as a complementary therapy to existing treatments like chemotherapy and radiation.[69]
- Minimal Side Effects:
- Traditional cancer treatments often come with significant side effects, affecting patients’ quality of life. Cold plasma therapy offers a less invasive and more targeted approach, with minimal side effects.[70]
- Patients undergoing cold plasma treatment have reported fewer adverse effects compared to conventional therapies, highlighting its potential as a preferable alternative or adjunctive treatment.[71]
- Integration with Other Treatments:
- Cold plasma therapy can be integrated with other cancer treatments to enhance overall efficacy. For example, combining cold plasma with chemotherapy or immunotherapy can potentially yield synergistic effects, improving cancer treatment outcomes.[72]
- Researchers are exploring the potential of cold plasma to sensitize cancer cells to chemotherapeutic agents, thereby enhancing the effectiveness of chemotherapy.[73]
The Mirari Cold Plasma device, with its unique nitric oxide-based cold plasma generation, may offer distinct advantages in cancer treatment applications. Nitric oxide has been shown to have anti-tumor effects, including the ability to induce apoptosis in cancer cells and inhibit tumor angiogenesis.[74] By leveraging the properties of nitric oxide, the Mirari device could potentially provide a targeted and effective approach to cancer therapy, minimizing damage to healthy tissues while maximizing the impact on tumor cells.[5]
Sterilization of Medical Equipment
Sterilization of medical equipment is a critical component of healthcare, and cold plasma technology provides an efficient and effective method for achieving high sterilization standards.[75]
- Broad-spectrum Antimicrobial Activity:
- Cold plasma exhibits broad-spectrum antimicrobial activity, effectively inactivating bacteria, viruses, fungi, and spores. This makes it an ideal solution for sterilizing a wide range of medical equipment, from surgical instruments to complex devices.[33]
- The reactive species generated during plasma discharge cause oxidative damage to microbial cells, leading to cell death and ensuring thorough sterilization.[69]
- Compatibility with Heat-sensitive Instruments:
- Many medical instruments are heat-sensitive and can be damaged by traditional high-temperature sterilization methods. Cold plasma offers a non-thermal alternative, making it suitable for sterilizing delicate instruments and devices.[76]
- For example, endoscopes and catheters, which are sensitive to heat and pressure, can be effectively sterilized using cold plasma without compromising their integrity.[77]
- Efficiency and Speed:
- Cold plasma sterilization processes are efficient and rapid, significantly reducing the time required for sterilization compared to traditional methods. This increased efficiency allows for quicker turnaround times in healthcare settings, enhancing overall operational workflow.[57]
- Studies have shown that cold plasma can achieve complete sterilization within minutes, making it a time-efficient option for medical facilities.[60]
- Environmental Benefits:
- Cold plasma sterilization is environmentally friendly, as it does not rely on harmful chemicals or produce hazardous waste. Traditional chemical sterilants like ethylene oxide pose environmental and health risks, whereas cold plasma offers a safer, greener alternative.[78]
- The use of atmospheric gases like air or argon in cold plasma sterilization systems eliminates the need for toxic chemicals, reducing environmental impact and enhancing sustainability.[79]
- Implementation in Healthcare Facilities:
- Healthcare facilities are increasingly incorporating cold plasma sterilization systems to enhance infection control practices. For example, plasma sterilization units are being installed in operating rooms, intensive care units, and outpatient clinics to ensure high levels of hygiene and safety.[80]
- The ease of integrating cold plasma systems into existing healthcare infrastructure further supports widespread adoption of this technology.[12]
Plasma-Assisted Therapy and Its Mechanisms
Plasma-assisted therapy encompasses a range of medical treatments that harness the power of cold plasma for therapeutic benefits. The underlying mechanisms involve complex interactions between reactive species and biological systems.[57]
![]() |
Oxidative Stress Induction:
Reactive oxygen and nitrogen species generated during cold plasma treatment induce oxidative stress in targeted cells. This oxidative stress leads to lipid peroxidation, protein denaturation, and DNA damage, resulting in cell apoptosis or necrosis.[69] The selective induction of oxidative stress makes cold plasma particularly effective in targeting pathogenic microorganisms and cancer cells while preserving healthy tissues.[27] |
![]() |
Cold plasma has been shown to modulate immune responses, enhancing the body’s natural defense mechanisms. The reactive species stimulate the release of cytokines and other immune mediators, promoting an anti-inflammatory response and accelerating tissue repair.[59]
Researchers are exploring the potential of cold plasma to modulate immune responses in autoimmune disorders and infections, providing new avenues for therapy.[82] |
![]() |
Cellular Regeneration and Repair:
Cold plasma stimulates cellular regeneration and repair processes, making it effective in wound healing and tissue regeneration. The reactive species activate signaling pathways that promote cell proliferation, migration, and differentiation.[61] In tissue engineering, cold plasma treatments are used to enhance the integration and functionality of tissue scaffolds and implants, supporting regenerative medicine applications.[83] |
![]() |
Electroporation:
Cold plasma treatment can induce electroporation in cellular membranes, increasing their permeability. This process facilitates the entry of therapeutic agents into cells, enhancing the delivery and efficacy of treatments.[84] Electroporation has been particularly useful in cancer therapy, where enhancing the uptake of chemotherapeutic agents into cancer cells can improve treatment outcomes.[73] |
![]() |
Applications in Chronic Conditions:
Plasma-assisted therapy is being explored for the treatment of chronic conditions such as non-healing wounds, inflammatory diseases, and infections. The ability to target chronic inflammation and promote tissue regeneration positions cold plasma as a promising therapeutic tool.[58] For example, clinical trials have demonstrated the effectiveness of cold plasma in treating chronic diabetic ulcers, reducing bacterial load, and promoting healing.[62] |
The Mirari Cold Plasma device, with its nitric oxide-based cold plasma generation, may offer distinct advantages in plasma-assisted therapy. Nitric oxide is known for its multiple biological roles, including vasodilation, immunomodulation, and promotion of angiogenesis.[85] By incorporating nitric oxide into the cold plasma treatment, the Mirari device could potentially enhance the therapeutic effects, particularly in applications such as wound healing, tissue regeneration, and chronic condition management.[5]
Cold Plasma for Musculoskeletal Disorders
Cold plasma technology shows promise in treating musculoskeletal disorders, offering innovative solutions for pain relief and tissue repair.[12]
- Tissue Regeneration and Repair:
- Cold plasma promotes tissue regeneration and repair by stimulating cellular activities essential for healing. The reactive species generated during treatment enhance the proliferation and differentiation of cells involved in tissue repair, such as chondrocytes and osteoblasts.[61]
- Studies have demonstrated improved healing outcomes in musculoskeletal injuries, such as tendonitis, ligament injuries, and bone fractures, following cold plasma treatment.[86]
- Reduction of Inflammation and Pain:
- The anti-inflammatory properties of cold plasma help reduce inflammation and pain associated with musculoskeletal disorders. By modulating immune responses and reducing the levels of pro-inflammatory cytokines, cold plasma treatment provides relief from chronic pain and inflammation.[59]
- Clinical applications have shown significant reductions in pain levels in patients with conditions like arthritis and tendonitis after cold plasma therapy.[87]
- Enhanced Rehabilitation:
- Cold plasma therapy can be integrated into rehabilitation programs to enhance recovery outcomes. By promoting faster tissue repair and reducing inflammation, cold plasma supports the rehabilitation process in patients with sports injuries and post-operative conditions.[88]
- For instance, athletes undergoing cold plasma treatment for tendon injuries have reported quicker recovery times and improved performance.[89]
- Potential for Non-Invasive Treatment:
- As a non-invasive treatment option, cold plasma offers a less risky and more patient-friendly alternative to surgical interventions. This makes it particularly appealing for treating musculoskeletal disorders that do not respond to conventional therapies.[55]
- Research is ongoing to explore cold plasma’s potential in treating conditions such as osteoarthritis, where traditional treatments have limited effectiveness.[90]
- Future Directions in Musculoskeletal Therapy:
- Ongoing research aims to optimize cold plasma delivery methods and understand its long-term effects on musculoskeletal tissues. Innovations in cold plasma device design and treatment protocols are expected to enhance its therapeutic potential further.[91]
- Future studies are focusing on exploring the combination of cold plasma with other regenerative therapies, such as stem cell treatments, to amplify its benefits in musculoskeletal repair.[92]
Cold Plasma in Agriculture
Cold plasma technology has emerged as a promising tool in agricultural applications, particularly in seed treatment, plant growth enhancement, and pathogen control. This approach utilizes cold atmospheric plasma (CAP), a novel technique that provides various reactive species conducive to improving plant health and yield.[94]
Seed Treatment and Plant Growth Enhancement
Cold plasma treatment of seeds and plants has proven effective in enhancing germination rates, plant vigor, and crop yields, contributing to sustainable agriculture practices.[95]
- Preparation of Seeds:
- Cold plasma treatment modifies the seed surface, enhancing water absorption and germination rates. Studies have shown that when seeds are treated with CAP, their physical properties change. For example, the surface becomes more porous, allowing for increased water uptake, which significantly accelerates germination.[96]
- Research indicated that plasma-treated seeds of various crops, including wheat and soybeans, exhibited increased root weight and height compared to untreated seeds. This growth enhancement is attributed to the activation of metabolic processes and the stimulation of phytohormones such as auxins and cytokinins, which are crucial for plant growth and development.[97]
- Mechanisms of Action:
- The efficacy of cold plasma in promoting plant growth is linked to the generation of reactive oxygen and nitrogen species during treatment. These species can enhance biochemical pathways that lead to improved seed vigor and growth rates, resulting in faster sprouting and healthier plants.[98]
- Studies suggest that plasma treatment can boost seed vigor, increase shoot length, and improve yield quality in various crops, making it a valuable tool for modern agriculture.[99]
- Optimization of Treatment Parameters:
- The optimization of treatment parameters such as gas type, exposure time, and power settings is crucial for achieving desired outcomes in seed treatment and plant growth enhancement. Tailoring these parameters to specific crop types and environmental conditions ensures maximum efficacy.[100]
- Applications in Different Crops:
- Cereal Crops: Cold plasma treatment has shown significant improvements in the germination and growth of cereal crops like wheat, rice, and maize. Enhanced root and shoot development contribute to higher yields and better crop resilience.[101]
- Fruits and Vegetables: In horticulture, cold plasma treatment improves the germination rates of fruit and vegetable seeds, leading to healthier and more robust plants. Studies have reported increased yields and improved quality of crops like tomatoes, cucumbers, and strawberries.[102]
- Legumes and Oilseeds: Plasma-treated legume and oilseed crops, such as soybeans and canola, exhibit improved seedling vigor and growth rates. The enhanced physiological responses contribute to higher productivity and better stress tolerance.[103]
Pathogen Control in Agriculture
Cold plasma technology serves as an effective pathogen control method in agriculture, providing a non-chemical approach to managing diseases and pests.[104]
![]() |
Disinfection Properties:
Cold plasma technology is recognized for its ability to disinfect plant surfaces and seeds. The reactive species produced during plasma treatment effectively kill a wide range of pathogens, including bacteria and fungi.[6] Studies have shown a significant reduction in microbial load on the surfaces of fruits and vegetables treated with CAP, promoting food safety and extending shelf life. For instance, plasma jet treatments have demonstrated efficacy against common human pathogens such as Salmonella and E. coli on various crops, including tomatoes and lettuce.[17] |
![]() |
Integration with Current Practices:
To maximize its effectiveness, cold plasma can be integrated with conventional agricultural practices, enhancing the existing methods of pest management and disease control. By applying plasma to seeds pre-sowing or to young plants, farmers can reduce the incidence of plant diseases while promoting growth.[98] This dual functionality makes cold plasma an innovative solution for sustainable farming practices, as it reduces reliance on chemical pesticides and fertilizers.[95] |
![]() |
Reduced Chemical Usage:
One of the significant benefits of cold plasma technology is its potential to reduce the use of chemical pesticides and fungicides. By providing an effective non-chemical method for pathogen control, cold plasma supports sustainable agriculture practices and minimizes chemical residues in the environment.[102] The reduction of chemical inputs aligns with global trends towards organic and eco-friendly farming practices.[53] |
![]() |
Applications in Different Crops:
Fruit Trees and Vineyards: Plasma treatment of fruit tree and vineyard crops can reduce the incidence of fungal diseases such as powdery mildew and botrytis, improving crop quality and yield.[105] Vegetables: Cold plasma effectively controls bacterial and fungal infections in vegetable crops like tomatoes, cucumbers, and lettuce, promoting healthy growth and reducing post-harvest losses.[32] Grains and Cereals: The disinfection of grain crops such as wheat and barley using cold plasma helps control seed-borne diseases, enhancing crop yield and quality.[100] |
Plasma-Activated Water for Irrigation
Plasma-activated water (PAW) is produced by treating water with cold plasma, creating a solution rich in reactive oxygen and nitrogen species that benefit plant growth and health.[106]
- Benefits for Plant Growth:
- PAW enhances seed germination and promotes plant development by triggering the release of endogenous defense hormones and improving plants’ immunity to diseases and stressors. Studies have demonstrated that PAW can enhance nutrient uptake and support vigorous growth.[107]
- The use of PAW for irrigation has shown improved growth rates and overall plant health compared to traditional irrigation methods.[108]
- Chemical-free and Eco-friendly:
- PAW offers an appealing alternative to conventional agricultural practices that rely on chemical fertilizers and pesticides. As a chemical-free and eco-friendly solution, PAW reduces the environmental impact of farming and supports sustainable agriculture.[95]
- The increased redox potential and conductivity of PAW contribute to improved soil health and nutrient availability for plants, without the need for chemical inputs.[95]
- Generation and Application:
- The generation of PAW involves the dissipation of cold atmospheric plasma in water, leading to an acidic environment with enhanced chemical properties. This method not only improves the water’s agricultural utility but also has applications in food safety and surface disinfection.[109]
- PAW can be easily integrated into existing irrigation systems, providing a seamless transition to more sustainable farming practices.[110]
- Applications in Different Crops:
- Field Crops: PAW irrigation has shown benefits in field crops such as corn, soybeans, and wheat, enhancing germination rates, plant vigor, and yield.[111]
- Greenhouse Crops: In greenhouse settings, PAW supports the growth of high-value crops like tomatoes, cucumbers, and leafy greens, promoting healthy and robust plants.[112]
- Orchards and Vineyards: The application of PAW in orchards and vineyards contributes to better fruit set, increased yields, and improved fruit quality.[3]
The Mirari Cold Plasma device, with its innovative nitric oxide-based cold plasma generation, may offer distinct advantages in the production of plasma-activated water for irrigation. Nitric oxide has been recognized for its role in plant signaling, growth regulation, and stress responses.[113] By incorporating nitric oxide into the plasma-activated water, the Mirari device could potentially enhance the beneficial effects of PAW on plant growth, health, and resilience.[5]
As the agricultural industry seeks sustainable and efficient solutions for crop irrigation, devices like Mirari are expected to play a crucial role. The integration of cold plasma technology with nitric oxide delivery in the generation of plasma-activated water opens up new possibilities for optimizing plant nutrition, promoting plant health, and supporting eco-friendly farming practices. Further research and collaboration between agricultural scientists, plant physiologists, and technology developers will be essential in fully realizing the potential of the Mirari Cold Plasma device and other similar innovations in revolutionizing irrigation practices in modern agriculture.[98]
Overall, cold plasma technology offers multiple advantages in agricultural applications, enhancing seed treatment, plant growth, pathogen control, and irrigation practices. By providing sustainable and eco-friendly alternatives, cold plasma technology supports the goal of achieving higher yields, better crop quality, and reduced environmental impact. Innovations like the Mirari Cold Plasma device, with its unique nitric oxide-based cold plasma generation, are expected to make significant contributions to the advancement of modern agriculture, offering targeted and effective solutions for various challenges faced by farmers and the agricultural industry as a whole.[94]
Industrial Applications of Cold Plasma
Cold plasma technology has gained significant traction in industrial applications, offering innovative solutions for material processing, surface treatment, energy production, and environmental protection. Its unique properties enable efficient and effective modifications without damaging the underlying materials.[7]
- Material Processing:
- Cold plasma is employed for cleaning, activation, and modification of surfaces. It effectively removes contaminants, etches surfaces, and enhances adhesion properties of coatings on various substrates such as metals, plastics, and ceramics.[114]
- Specific parameters such as gas composition, voltage, and treatment duration can be tailored to achieve desired outcomes, including improved mechanical, chemical, and optical properties of treated surfaces.[8]
- Surface Treatment:
- Applications include improving the wettability of surfaces, increasing adhesion for coatings, and creating biocompatible layers on implantable devices. These treatments are particularly valuable in industries like aerospace, automotive, and biomedical, where enhanced surface characteristics are critical for performance and longevity.[115]
- Cold plasma technology facilitates coating processes by promoting better adhesion between coatings and substrates, leading to improved durability and performance of final products.[29]
- Energy Production:
- In energy production, cold plasma enhances processes such as biomass conversion, improving efficiency and output. For example, plasma-assisted gasification of biomass can produce syngas with higher energy content.[116]
- Cold plasma also has potential applications in hydrogen production, catalyzing reactions that produce hydrogen from water or hydrocarbons.[117]
- Environmental Protection:
- Cold plasma is effective in treating wastewater, degrading pollutants, and inactivating pathogens, contributing to sustainable practices and reducing environmental impact. Plasma treatment can break down complex organic pollutants into simpler, less harmful compounds.[118]
- Cold plasma technology is also used in air purification systems to remove contaminants and pathogens from indoor environments, improving air quality and safety.[119]
Cold Plasma in Material Processing
Cold plasma technology plays a critical role in material processing, offering efficient and effective methods for surface treatment and modification.[7]
- Cleaning and Activation:
- Cold plasma effectively cleans and activates surfaces, removing organic and inorganic contaminants. This process can be used to prepare surfaces for subsequent treatments like coating or bonding.[120]
- By creating a highly reactive surface, cold plasma improves the adhesion of coatings and enhances the performance of adhesive joints.[29]
- Etching and Patterning:
- Cold plasma is used for precise etching and patterning of surfaces, particularly in semiconductor and microelectronics industries. It enables the creation of fine patterns and structures on substrates with high precision, essential for fabricating electronic components and devices.[25] The plasma etching process selectively removes material from specific areas, guided by patterning masks, resulting in the desired microstructures for applications in integrated circuits, sensors, and microelectromechanical systems (MEMS).[28]
Surface Treatment and Coating Technologies
Cold plasma technology offers advanced surface treatment and coating solutions, transforming the properties of materials to meet specific industry requirements. Here’s a detailed examination of these applications:
- Surface Functionalization:
- Improving Adhesion: Cold plasma treatments can enhance the adhesion properties of surfaces, which is vital for coatings, paints, and adhesives. By altering the surface energy and introducing functional groups, cold plasma ensures better bonding between substrates and coatings.[115]
- Tailoring Surface Properties: Cold plasma can be used to tailor the wettability and hydrophobicity of surfaces. For example, treating polymer surfaces with oxygen plasma enhances wettability, making them more suitable for subsequent coating applications.[120]
- Enhanced Coating Processes:
- Barrier Coatings: Cold plasma technology is employed to apply barrier coatings on packaging materials, enhancing their resistance to gases and moisture. This application is crucial in food packaging, where prolonging shelf life and maintaining quality are paramount.[48]
- Protective Coatings: Applying protective coatings using cold plasma can improve the durability and resistance of materials. These coatings protect against corrosion, wear, and environmental degradation, making them essential in industries such as automotive and construction.[114]
- Biomedical Applications:
- Biocompatible Coatings: In biomedical engineering, cold plasma is used to apply biocompatible coatings on medical implants and devices. These coatings improve the integration and performance of implants within the human body, reducing rejection and promoting healing.[115]
- Antimicrobial Coatings: Cold plasma treatments can introduce antimicrobial properties to surfaces, preventing biofilm formation and microbial growth. This application is significant in healthcare settings, where maintaining sterility and preventing infections are critical.[116]
- Nanocoatings:
- Thin-Film Deposition: Cold plasma facilitates the deposition of ultra-thin films with precise control over thickness and composition. These nanocoatings can significantly enhance the optical, electrical, and mechanical properties of substrates, finding applications in electronics, optics, and protective films.[51]
- Functional Nanostructures: Cold plasma technology allows the fabrication of nanostructured coatings that provide unique functionalities, such as anti-reflective properties or enhanced catalytic activity. These innovative coatings are used in solar cells, sensors, and catalytic converters.[52]
- Environmental Benefits:
- Eco-Friendly Processes: Cold plasma coating and surface treatment processes are environmentally friendly, as they often replace chemical-intensive methods. This reduction in chemical usage minimizes hazardous waste and reduces the environmental footprint of manufacturing processes.[118]
- Energy Efficiency: Cold plasma processes are energy-efficient, requiring lower temperatures and shorter treatment times compared to conventional methods. This efficiency translates into cost savings and reduced energy consumption.[119]
Role in Energy Production and Environmental Protection
Cold plasma technology plays an instrumental role in advancing energy production and promoting environmental sustainability through diverse applications.
- Energy Production:
- Biomass Conversion: Cold plasma enhances biomass conversion processes, such as gasification and pyrolysis, producing cleaner and more efficient energy sources. Plasma-assisted biomass gasification generates syngas with higher calorific value, improving energy yield and reducing waste.[95]
- Hydrogen Production: Cold plasma technologies are explored for their potential in hydrogen production. Plasma-induced reactions can split water molecules or reform hydrocarbons to produce hydrogen, contributing to clean energy initiatives.[98]
- Fuel Cells: Plasma treatments enhance the performance of fuel cell components, such as electrodes and membranes. By improving surface properties and catalytic activity, cold plasma can increase the efficiency and durability of fuel cells used in clean energy systems.[115]
- Environmental Protection:
- Wastewater Treatment: Cold plasma is effective in treating wastewater by breaking down pollutants and inactivating pathogens. The reactive species generated by plasma can degrade complex organic compounds into harmless substances, improving water quality and safety.[118]
- Air Purification: Cold plasma technology is used in air purification systems to remove contaminants, VOCs (volatile organic compounds), and pathogens from indoor air. Plasma-induced reactions neutralize harmful pollutants, enhancing air quality in residential, commercial, and industrial environments.[119]
- Soil Remediation: Plasma treatments can be employed in soil remediation processes to degrade organic pollutants and detoxify contaminated soils. This application is crucial for restoring polluted lands and promoting sustainable land use.[112]
- Reduction of Greenhouse Gas Emissions:
- Carbon Capture and Utilization: Cold plasma technologies are explored for capturing and converting CO2 into value-added products. Plasma-catalyzed reactions can transform CO2 into useful chemicals, reducing its environmental impact and contributing to circular economy practices.[19]
- Methane Oxidation: Cold plasma can oxidize methane, a potent greenhouse gas, to less harmful compounds. This application is significant in mitigating methane emissions from sources such as agriculture and landfills.[20]
- Sustainable Manufacturing:
- Green Chemistry: Cold plasma enables sustainable manufacturing practices by reducing or eliminating the need for harmful chemicals in production processes. Plasma-assisted reactions offer cleaner and more efficient alternatives for chemical synthesis and material processing.[21]
- Resource Efficiency: Plasma processes often require fewer raw materials and generate less waste compared to conventional methods. This efficiency supports resource conservation and reduces the environmental footprint of industrial operations.[22]
Emerging Trends and Research Directions
Recent innovations and interdisciplinary approaches continue to expand the horizons of cold plasma technology, unlocking new applications and enhancing its efficiency.
- Recent Innovations:
- Automated Plasma Systems: Advances in automation are leading to the development of integrated plasma systems that enhance process efficiency and reliability. These systems incorporate real-time monitoring and control, optimizing plasma parameters for consistent and effective treatments.[4]
- Portable Plasma Devices: The development of portable and handheld plasma devices is opening up new applications in field settings, such as on-site disinfection, medical treatments, and agricultural interventions.[10]
- Multidisciplinary Approaches:
- Interdisciplinary Research Teams: Collaborative efforts between physicists, chemists, engineers, and biologists are driving advancements in cold plasma technology. These multidisciplinary teams enable a holistic understanding of plasma interactions and foster innovative solutions.[11]
- Combining Technologies: Integrating cold plasma with other emerging technologies, such as nanotechnology, biotechnology, and artificial intelligence, is creating synergistic effects and enhancing the overall impact of plasma applications.[12]
- Future Prospects:
- Smart Agriculture: Cold plasma technology is poised to play a key role in smart agriculture, providing precision treatments for seeds, crops, and soil. Integration with IoT (Internet of Things) and data analytics will enable tailored plasma treatments that optimize agricultural productivity and sustainability.[98]
- Next-Generation Medical Devices: Advances in cold plasma will lead to the development of next-generation medical devices that offer non-invasive, effective, and patient-friendly treatments for various conditions.[56]
- Circular Economy: Plasma technologies will contribute to circular economy practices by transforming waste materials into valuable resources and reducing environmental impact. Research is focusing on scalable solutions for waste management and resource recovery using plasma-assisted processes.[118]
Challenges and limitations of cold plasma technology
Despite its numerous advantages, cold plasma technology faces several challenges and limitations that must be addressed to realize its full potential.
- Technical Challenges in Implementation:
- Uniformity of Treatment: Achieving uniform treatment effects across different substrates and materials poses a significant challenge. Variability in plasma distribution can result in inconsistent outcomes, affecting the efficacy of treatments.[18]
- Control of Reactive Species: The generation and control of reactive species are critical for effective plasma treatments. Variability in operating conditions can lead to unpredictable results, necessitating precise control and calibration of plasma parameters.[69]
- Scalability: Scaling up cold plasma processes for industrial applications requires addressing challenges related to reactor design, energy efficiency, and process integration.[20]
- Economic Considerations and Feasibility:
- High Initial Costs: The capital investment required for cold plasma equipment and infrastructure can be prohibitive, particularly for small and medium-sized enterprises (SMEs). The cost of specialized equipment and the need for skilled personnel add to the economic burden.[38]
- Operational Costs: The ongoing operational expenses, including energy consumption and maintenance, can be significant. A thorough economic analysis is necessary to determine the return on investment and cost-effectiveness of plasma technologies compared to conventional methods.[43]
- Environmental Impact Assessments:
- Energy Consumption: Although cold plasma processes are energy-efficient, the actual energy costs and carbon footprint must be carefully evaluated. Transitioning to renewable energy sources can enhance the sustainability of plasma technologies.[119]
- Regulatory and Safety Considerations: The lack of standardized regulations and safety guidelines for cold plasma applications poses challenges for commercialization. Comprehensive risk assessments and the formulation of robust safety protocols are essential.[79]
Conclusion and Future Directions
Cold plasma technology represents a transformative approach with wide-ranging applications across various industries. Its ability to provide non-thermal, efficient, and environmentally friendly solutions marks it as a key player in addressing contemporary challenges.[11]
Summary of Key Applications
Cold plasma technology has demonstrated its versatility and effectiveness in numerous applications, including:
- Food Industry:
- Medical Sector:
- Agriculture:
- Industrial Applications:
- Material Processing: Cleaning, activating, and modifying surfaces for better performance.[115]
- Surface Coatings: Applying protective and functional coatings to enhance material properties.[51]
- Environmental Protection: Treating wastewater, air purification, and reducing greenhouse gas emissions.[118]
The Mirari Cold Plasma device, with its innovative nitric oxide-based cold plasma generation, has the potential to significantly contribute to these key applications. The unique properties of nitric oxide, combined with the versatility of cold plasma technology, position the Mirari device as a powerful tool for addressing challenges in food safety, medical treatments, agricultural productivity, and industrial processes.[5] As research continues to explore the specific benefits and mechanisms of nitric oxide-enriched cold plasma, the Mirari device is expected to drive advancements and open up new possibilities across these diverse sectors.[66]
The Future of Cold Plasma Technology in Various Sectors
As cold plasma technology continues to evolve, its future prospects across different sectors appear promising:
- Integration into Automated Systems:
- Developing automated plasma systems will enhance efficiency and consistency, making cold plasma more accessible and practical for widespread industrial applications.[4]
- Expanding Applications:
- Ongoing research and innovations will expand the scope of plasma applications, incorporating it into emerging fields such as smart agriculture, advanced medical therapies, and sustainable manufacturing practices.[11]
- Technological Advancements:
- Innovations in plasma generation methods, device design, and process optimization will improve the efficacy and cost-effectiveness of cold plasma technologies.[18]
- Environmental Sustainability:
- As global awareness of environmental issues grows, cold plasma’s eco-friendly profile positions it as a leading solution in addressing challenges related to waste management, resource conservation, and sustainable agriculture.[118]
- Collaborative Research:
- Interdisciplinary and collaborative research efforts will drive the development of innovative solutions, combining cold plasma with other cutting-edge technologies to create synergistic effects.[12]
The Mirari Cold Plasma device, with its nitric oxide-based cold plasma generation, is well-positioned to play a significant role in shaping the future of cold plasma technology across various sectors.[5] The integration of nitric oxide delivery into automated plasma systems and portable devices could revolutionize the way treatments are administered, enabling precise and targeted interventions in healthcare, agriculture, and industrial settings.[66]
Moreover, the expanding applications of the Mirari device, driven by ongoing research and collaborations, are expected to unlock new frontiers in fields such as regenerative medicine, precision agriculture, and sustainable manufacturing.[98] The unique combination of cold plasma and nitric oxide delivery offered by the Mirari device has the potential to address complex challenges and provide innovative solutions that benefit human health, food security, and environmental sustainability.[67]
As technological advancements continue to refine the capabilities of the Mirari Cold Plasma device, its future prospects appear bright. The device’s eco-friendly profile, coupled with its versatility and effectiveness, positions it as a key player in the transition towards more sustainable and efficient practices across various industries.[43] With ongoing research and development, the Mirari device is poised to make significant contributions to the creation of smart, sustainable, and resilient systems that address the pressing challenges of our time.[2]
In conclusion, cold plasma technology holds the potential to revolutionize various industries by providing effective, sustainable, and innovative solutions. The Mirari Cold Plasma device, with its nitric oxide-based cold plasma generation, represents a significant advancement in this field, offering a powerful tool for transforming healthcare, agriculture, industrial processes, and environmental protection.[1] As research progresses and collaborations deepen, the future of cold plasma technology, and the Mirari device in particular, appears promising, with the potential to shape a more sustainable, healthier, and technologically advanced world. Embracing the challenges and opportunities that lie ahead, the Mirari Cold Plasma device is set to play a pivotal role in driving the widespread adoption and impact of cold plasma technology across diverse sectors, ultimately benefiting society and the planet as a whole.
References
- Fridman, G., Friedman, G., Gutsol, A., Shekhter, A. B., Vasilets, V. N., & Fridman, A. (2008). Applied plasma medicine. Plasma Processes and Polymers, 5(6), 503-533. https://doi.org/10.1002/ppap.200700154
- Laroussi, M. (2018). Plasma medicine: A brief introduction. Plasma, 1(1), 47-60. https://doi.org/10.3390/plasma1010005
- Schlüter, O., Ehlbeck, J., Hertel, C., Habermeyer, M., Roth, A., Engel, K. H., Holzhauser, T., Knorr, D., & Eisenbrand, G. (2013). Opinion on the use of plasma processes for treatment of foods. Molecular Nutrition & Food Research, 57(5), 920-927. https://doi.org/10.1002/mnfr.201300039
- Lu, X., Naidis, G. V., Laroussi, M., Reuter, S., Graves, D. B., & Ostrikov, K. (2016). Reactive species in non-equilibrium atmospheric-pressure plasmas: Generation, transport, and biological effects. Physics Reports, 630, 1-84. https://doi.org/10.1016/j.physrep.2016.03.003
- General Vibronics. (2024). Mirari Cold Plasma Device. Retrieved September 22, 2024, from https://miraridoctor.com/product/
- Bernhardt, T., Semmler, M. L., Schäfer, M., Bekeschus, S., Emmert, S., & Boeckmann, L. (2019). Plasma Medicine: Applications of Cold Atmospheric Pressure Plasma in Dermatology. Oxidative Medicine and Cellular Longevity, 2019, 3873928. https://doi.org/10.1155/2019/3873928
- Tendero, C., Tixier, C., Tristant, P., Desmaison, J., & Leprince, P. (2006). Atmospheric pressure plasmas: A review. Spectrochimica Acta Part B: Atomic Spectroscopy, 61(1), 2-30. https://doi.org/10.1016/j.sab.2005.10.003
- Fridman, A. (2008). Plasma Chemistry. Cambridge University Press. https://doi.org/10.1017/CBO9780511546075
- Pankaj, S. K., Bueno-Ferrer, C., Misra, N. N., Milosavljević, V., O’Donnell, C. P., Bourke, P., Keener, K. M., & Cullen, P. J. (2014). Applications of cold plasma technology in food packaging. Trends in Food Science & Technology, 35(1), 5-17. https://doi.org/10.1016/j.tifs.2013.10.009
- Laroussi, M., & Akan, T. (2007). Arc-free atmospheric pressure cold plasma jets: A review. Plasma Processes and Polymers, 4(9), 777-788. https://doi.org/10.1002/ppap.200700066
- Adamovich, I., Baalrud, S. D., Bogaerts, A., Bruggeman, P. J., Cappelli, M., Colombo, V., … & Vardelle, A. (2017). The 2017 Plasma Roadmap: Low temperature plasma science and technology. Journal of Physics D: Applied Physics, 50(32), 323001. https://doi.org/10.1088/1361-6463/aa76f5
- Weltmann, K. D., & von Woedtke, T. (2017). Plasma medicine—current state of research and medical application. Plasma Physics and Controlled Fusion, 59(1), 014031. https://doi.org/10.1088/0741-3335/59/1/014031
- Bruggeman, P. J., Kushner, M. J., Locke, B. R., Gardeniers, J. G. E., Graham, W. G., Graves, D. B., … & Zvereva, G. (2016). Plasma–liquid interactions: a review and roadmap. Plasma Sources Science and Technology, 25(5), 053002. https://doi.org/10.1088/0963-0252/25/5/053002
- Niemira, B. A. (2012). Cold plasma decontamination of foods. Annual Review of Food Science and Technology, 3, 125-142. https://doi.org/10.1146/annurev-food-022811-101132
- Stoffels, E., Sakiyama, Y., & Graves, D. B. (2008). Cold atmospheric plasma: charged species and their interactions with cells and tissues. IEEE Transactions on Plasma Science, 36(4), 1441-1457. https://doi.org/10.1109/TPS.2008.2001084
- Bourke, P., Ziuzina, D., Boehm, D., Cullen, P. J., & Keener, K. (2018). The potential of cold plasma for safe and sustainable food production. Trends in Biotechnology, 36(6), 615-626. https://doi.org/10.1016/j.tibtech.2017.11.001
- Ziuzina, D., Patil, S., Cullen, P. J., Keener, K. M., & Bourke, P. (2014). Atmospheric cold plasma inactivation of Escherichia coli, Salmonella enterica serovar Typhimurium and Listeria monocytogenes inoculated on fresh produce. Food Microbiology, 42, 109-116. https://doi.org/10.1016/j.fm.2014.02.007
- Laroussi, M., Lu, X., & Keidar, M. (2017). Perspective: The physics, diagnostics, and applications of atmospheric pressure low temperature plasma sources used in plasma medicine. Journal of Applied Physics, 122(2), 020901. https://doi.org/10.1063/1.4993710
- Bogaerts, A., Neyts, E., Gijbels, R., & van der Mullen, J. (2002). Gas discharge plasmas and their applications. Spectrochimica Acta Part B: Atomic Spectroscopy, 57(4), 609-658. https://doi.org/10.1016/S0584-8547(01)00406-2
- Kogelschatz, U. (2003). Dielectric-barrier discharges: Their history, discharge physics, and industrial applications. Plasma Chemistry and Plasma Processing, 23(1), 1-46. https://doi.org/10.1023/A:1022470901385
- Bárdos, L., & Baránková, H. (2010). Cold atmospheric plasma: Sources, processes, and applications. Thin Solid Films, 518(23), 6705-6713. https://doi.org/10.1016/j.tsf.2010.07.044
- Moisan, M., Barbeau, J., Crevier, M. C., Pelletier, J., Philip, N., & Saoudi, B. (2002). Plasma sterilization. Methods and mechanisms. Pure and Applied Chemistry, 74(3), 349-358. https://doi.org/10.1351/pac200274030349
- Lu, X., Laroussi, M., & Puech, V. (2012). On atmospheric-pressure non-equilibrium plasma jets and plasma bullets. Plasma Sources Science and Technology, 21(3), 034005. https://doi.org/10.1088/0963-0252/21/3/034005
- Misra, N. N., Tiwari, B. K., Raghavarao, K. S. M. S., & Cullen, P. J. (2011). Nonthermal plasma inactivation of food-borne pathogens. Food Engineering Reviews, 3(3-4), 159-170. https://doi.org/10.1007/s12393-011-9041-9
- Lieberman, M. A., & Lichtenberg, A. J. (2005). Principles of plasma discharges and materials processing. John Wiley & Sons. https://doi.org/10.1002/0471724254
- Denes, F. S., & Manolache, S. (2004). Macromolecular plasma-chemistry: an emerging field of polymer science. Progress in Polymer Science, 29(8), 815-885. https://doi.org/10.1016/j.progpolymsci.2004.05.001
- Keidar, M., Shashurin, A., Volotskova, O., Ann Stepp, M., Srinivasan, P., Sandler, A., & Trink, B. (2013). Cold atmospheric plasma in cancer therapy. Physics of Plasmas, 20(5), 057101. https://doi.org/10.1063/1.4801516
- Donnelly, V. M., & Kornblit, A. (2013). Plasma etching: Yesterday, today, and tomorrow. Journal of Vacuum Science & Technology A: Vacuum, Surfaces, and Films, 31(5), 050825. https://doi.org/10.1116/1.4819316
- Hegemann, D., Brunner, H., & Oehr, C. (2003). Plasma treatment of polymers for surface and adhesion improvement. Nuclear Instruments and Methods in Physics Research Section B: Beam Interactions with Materials and Atoms, 208, 281-286. https://doi.org/10.1016/S0168-583X(03)00644-X
- Tappi, S., Berardinelli, A., Ragni, L., Dalla Rosa, M., Guarnieri, A., & Rocculi, P. (2014). Atmospheric gas plasma treatment of fresh-cut apples. Innovative Food Science & Emerging Technologies, 21, 114-122. https://doi.org/10.1016/j.ifset.2013.09.012
- ten Bosch, L., Pfohl, K., Avramidis, G., Wieneke, S., Viöl, W., & Karlovsky, P. (2017). Plasma-Based Degradation of Mycotoxins Produced by Fusarium, Aspergillus and Alternaria Species. Toxins, 9(3), 97. https://doi.org/10.3390/toxins9030097
- Misra, N. N., Keener, K. M., Bourke, P., Mosnier, J. P., & Cullen, P. J. (2014). In-package atmospheric pressure cold plasma treatment of cherry tomatoes. Journal of Bioscience and Bioengineering, 118(2), 177-182. https://doi.org/10.1016/j.jbiosc.2014.02.005
- Laroussi, M. (2005). Low temperature plasma-based sterilization: Overview and state-of-the-art. Plasma Processes and Polymers, 2(5), 391-400. https://doi.org/10.1002/ppap.200400078
- Pankaj, S. K., Misra, N. N., & Cullen, P. J. (2013). Kinetics of tomato peroxidase inactivation by atmospheric pressure cold plasma based on dielectric barrier discharge. Innovative Food Science & Emerging Technologies, 19, 153-157. https://doi.org/10.1016/j.ifset.2013.03.001
- Misra, N. N., Patil, S., Moiseev, T., Bourke, P., Mosnier, J. P., Keener, K. M., & Cullen, P. J. (2014). In-package atmospheric pressure cold plasma treatment of strawberries. Journal of Food Engineering, 125, 131-138. https://doi.org/10.1016/j.jfoodeng.2013.10.023
- Noriega, E., Shama, G., Laca, A., Díaz, M., & Kong, M. G. (2011). Cold atmospheric gas plasma disinfection of chicken meat and chicken skin contaminated with Listeria innocua. Food Microbiology, 28(7), 1293-1300. https://doi.org/10.1016/j.fm.2011.05.007
- Gurol, C., Ekinci, F. Y., Aslan, N., & Korachi, M. (2012). Low temperature plasma for decontamination of E. coli in milk. International Journal of Food Microbiology, 157(1), 1-5. https://doi.org/10.1016/j.ijfoodmicro.2012.02.016
- Pankaj, S. K., & Keener, K. M. (2017). Cold plasma: background, applications and current trends. Current Opinion in Food Science, 16, 49-52. https://doi.org/10.1016/j.cofs.2017.07.008
- Ramazzina, I., Berardinelli, A., Rizzi, F., Tappi, S., Ragni, L., Sacchetti, G., & Rocculi, P. (2015). Effect of cold plasma treatment on physico-chemical parameters and antioxidant activity of minimally processed kiwifruit. Postharvest Biology and Technology, 107, 55-65. https://doi.org/10.1016/j.postharvbio.2015.04.008
- Segat, A., Misra, N. N., Cullen, P. J., & Innocente, N. (2015). Atmospheric pressure cold plasma (ACP) treatment of whey protein isolate model solution. Innovative Food Science & Emerging Technologies, 29, 247-254. https://doi.org/10.1016/j.ifset.2015.03.014
- Hojnik, N., Cvelbar, U., Tavčar-Kalcher, G., Walsh, J. L., & Križaj, I. (2017). Mycotoxin decontamination of food: Cold atmospheric pressure plasma versus “classic” decontamination. Toxins, 9(5), 151. https://doi.org/10.3390/toxins9050151
- Devi, Y., Thirumdas, R., Sarangapani, C., Deshmukh, R. R., & Annapure, U. S. (2017). Influence of cold plasma on fungal growth and aflatoxins production on groundnuts. Food Control, 77, 187-191. https://doi.org/10.1016/j.foodcont.2017.02.019
- Misra, N. N., Pankaj, S. K., Segat, A., & Ishikawa, K. (2016). Cold plasma interactions with enzymes in foods and model systems. Trends in Food Science & Technology, 55, 39-47. https://doi.org/10.1016/j.tifs.2016.07.001
- Gavahian, M., & Khaneghah, A. M. (2020). Cold plasma as a tool for the elimination of food contaminants: Recent advances and future trends. Critical Reviews in Food Science and Nutrition, 60(9), 1581-1592. https://doi.org/10.1080/10408398.2019.1584600
- Dufour, T., Poncin-Epaillard, F., & Desbrieres, J. (2017). Surface modification of chitosan membranes by low pressure plasmas: Effect of operating parameters. Carbohydrate Polymers, 177, 109-118. https://doi.org/10.1016/j.carbpol.2017.08.097
- Ozdemir, M., Yurteri, C. U., & Sadikoglu, H. (1999). Physical polymer surface modification methods and applications in food packaging polymers. Critical Reviews in Food Science and Nutrition, 39(5), 457-477. https://doi.org/10.1080/10408699991279240
- Loo, C. Y., Young, P. M., Lee, W. H., Cavaliere, R., Whitchurch, C. B., & Rohanizadeh, R. (2012). Superhydrophobic, nanotextured polyvinyl chloride films for delaying Pseudomonas aeruginosa attachment to intubation tubes and medical plastics. Acta Biomaterialia, 8(5), 1881-1890. https://doi.org/10.1016/j.actbio.2012.01.015
- Pankaj, S. K., Bueno-Ferrer, C., Misra, N. N., O’Neill, L., Jiménez, A., Bourke, P., & Cullen, P. J. (2014). Characterization of polylactic acid films for food packaging as affected by dielectric barrier discharge atmospheric plasma. Innovative Food Science & Emerging Technologies, 21, 107-113. https://doi.org/10.1016/j.ifset.2013.10.007
- Soni, K. A., & Nannapaneni, R. (2010). Removal of Listeria monocytogenes biofilms with bacteriophage P100. Journal of Food Protection, 73(8), 1519-1524. https://doi.org/10.4315/0362-028X-73.8.1519
- Traba, C., & Liang, J. F. (2011). Susceptibility of Staphylococcus aureus biofilms to reactive discharge gases. Biofouling, 27(7), 763-772. https://doi.org/10.1080/08927014.2011.602188
- Morent, R., De Geyter, N., Desmet, T., Dubruel, P., & Leys, C. (2011). Plasma surface modification of biodegradable polymers: A review. Plasma Processes and Polymers, 8(3), 171-190. https://doi.org/10.1002/ppap.201000153
- Wu, J., Wu, J., Huang, Y., Liu, Q., He, P., & Zhou, M. (2021). Z-scheme heterojunction of flower microsphere Bi7O9I3 surface loaded with gray TiO2 particles for photocatalytic oxidation of gas-phase Hg0. Applied Surface Science, 567, 149240. https://doi.org/10.1016/j.apsusc.2021.149240
- Mandal, R., Singh, A., & Singh, A. P. (2018). Recent developments in cold plasma decontamination technology in the food industry. Trends in Food Science & Technology, 80, 93-103. https://doi.org/10.1016/j.tifs.2018.07.014
- Chaiwong, C., Rachtanapun, P., Wongchaiya, P., Auras, R., & Boonyawan, D. (2010). Effect of plasma treatment on hydrophobicity and barrier property of polylactic acid. Surface and Coatings Technology, 204(18-19), 2933-2939. https://doi.org/10.1016/j.surfcoat.2010.02.048
- Isbary, G., Shimizu, T., Li, Y. F., Stolz, W., Thomas, H. M., Morfill, G. E., & Zimmermann, J. L. (2013). Cold atmospheric plasma devices for medical issues. Expert Review of Medical Devices, 10(3), 367-377. https://doi.org/10.1586/erd.13.4
- Metelmann, H. R., Seebauer, C., Miller, V., Fridman, A., Bauer, G., Graves, D. B., … & von Woedtke, T. (2018). Clinical experience with cold plasma in the treatment of locally advanced head and neck cancer. Clinical Plasma Medicine, 9, 6-13. https://doi.org/10.1016/j.cpme.2017.09.001
- Kong, M. G., Kroesen, G., Morfill, G., Nosenko, T., Shimizu, T., Van Dijk, J., & Zimmermann, J. L. (2009). Plasma medicine: an introductory review. New Journal of Physics, 11(11), 115012. https://doi.org/10.1088/1367-2630/11/11/115012
- Isbary, G., Heinlin, J., Shimizu, T., Zimmermann, J. L., Morfill, G., Schmidt, H. U., … & Karrer, S. (2012). Successful and safe use of 2 min cold atmospheric argon plasma in chronic wounds: results of a randomized controlled trial. British Journal of Dermatology, 167(2), 404-410. https://doi.org/10.1111/j.1365-2133.2012.10923.x
- Bekeschus, S., Schmidt, A., Weltmann, K. D., & von Woedtke, T. (2016). The plasma jet kINPen – A powerful tool for wound healing. Clinical Plasma Medicine, 4(1), 19-28. https://doi.org/10.1016/j.cpme.2016.01.001
- Isbary, G., Morfill, G., Schmidt, H. U., Georgi, M., Ramrath, K., Heinlin, J., … & Shimizu, T. (2010). A first prospective randomized controlled trial to decrease bacterial load using cold atmospheric argon plasma on chronic wounds in patients. British Journal of Dermatology, 163(1), 78-82. https://doi.org/10.1111/j.1365-2133.2010.09744.x
- Arndt, S., Unger, P., Wacker, E., Shimizu, T., Heinlin, J., Li, Y. F., … & Morfill, G. E. (2013). Cold atmospheric plasma (CAP) changes gene expression of key molecules of the wound healing machinery and improves wound healing in vitro and in vivo. PloS one, 8(11), e79325. https://doi.org/10.1371/journal.pone.0079325
- Stratmann, B., Costea, T. C., Nolte, C., Hiller, J., Schmidt, J., Reindel, J., … & Tschoepe, D. (2020). Effect of cold atmospheric plasma therapy vs standard therapy placebo on wound healing in patients with diabetic foot ulcers: a randomized clinical trial. JAMA network open, 3(7), e2010411-e2010411. https://doi.org/10.1001/jamanetworkopen.2020.10411
- Kalghatgi, S., Friedman, G., Fridman, A., & Clyne, A. M. (2010). Endothelial cell proliferation is enhanced by low dose non-thermal plasma through fibroblast growth factor-2 release. Annals of biomedical engineering, 38(3), 748-757. https://doi.org/10.1007/s10439-009-9868-x
- Heinlin, J., Isbary, G., Stolz, W., Morfill, G., Landthaler, M., Shimizu, T., … & Karrer, S. (2011). Plasma applications in medicine with a special focus on dermatology. Journal of the European Academy of Dermatology and Venereology, 25(1), 1-11. https://doi.org/10.1111/j.1468-3083.2010.03702.x
- Ulrich, C., Kluschke, F., Patzelt, A., Vandersee, S., Czaika, V. A., Richter, H., … & Lange-Asschenfeldt, B. (2015). Clinical use of cold atmospheric pressure argon plasma in chronic leg ulcers: A pilot study. Journal of wound care, 24(5), 196-203. https://doi.org/10.12968/jowc.2015.24.5.196
- Luo, J. D., & Chen, A. F. (2005). Nitric oxide: a newly discovered function on wound healing. Acta Pharmacologica Sinica, 26(3), 259-264. https://doi.org/10.1111/j.1745-7254.2005.00058.x
- Keidar, M., Walk, R., Shashurin, A., Srinivasan, P., Sandler, A., Dasgupta, S., … & Trink, B. (2011). Cold plasma selectivity and the possibility of a paradigm shift in cancer therapy. British journal of cancer, 105(9), 1295-1301. https://doi.org/10.1038/bjc.2011.386
- Yan, D., Sherman, J. H., & Keidar, M. (2017). Cold atmospheric plasma, a novel promising anti-cancer treatment modality. Oncotarget, 8(9), 15977-15995. https://doi.org/10.18632/oncotarget.13304
- Graves, D. B. (2014). The emerging role of reactive oxygen and nitrogen species in redox biology and some implications for plasma applications to medicine and biology. Journal of Physics D: Applied Physics, 45(26), 263001. https://doi.org/10.1088/0022-3727/45/26/263001
- Hoffmann, C., Berganza, C., & Zhang, J. (2013). Cold Atmospheric Plasma: methods of production and application in dentistry and oncology. Medical gas research, 3(1), 21. https://doi.org/10.1186/2045-9912-3-21
- Schuster, M., Seebauer, C., Rutkowski, R., Hauschild, A., Podmelle, F., Metelmann, C., … & Metelmann, H. R. (2016). Visible tumor surface response to physical plasma and apoptotic cell kill in head and neck cancer. Journal of Cranio-Maxillofacial Surgery, 44(9), 1445-1452. https://doi.org/10.1016/j.jcms.2016.07.001
- Bauer, G., & Graves, D. B. (2016). Mechanisms of selective antitumor action of cold atmospheric plasma-derived reactive oxygen and nitrogen species. Plasma Processes and Polymers, 13(12), 1157-1178. https://doi.org/10.1002/ppap.201600089
- Chung, W. H. (2016). Mechanisms of a novel anticancer therapeutic strategy involving atmospheric pressure plasma-mediated apoptosis and DNA strand break formation. Archives of pharmacal research, 39(1), 1-9. https://doi.org/10.1007/s12272-015-0644-1
- Fukumura, D., Kashiwagi, S., & Jain, R. K. (2006). The role of nitric oxide in tumour progression. Nature Reviews Cancer, 6(7), 521-534. https://doi.org/10.1038/nrc1910
- Shintani, H., Sakudo, A., Burke, P., & McDonnell, G. (2010). Gas plasma sterilization of microorganisms and mechanisms of action. Experimental and therapeutic medicine, 1(5), 731-738. https://doi.org/10.3892/etm.2010.136
- Morfill, G. E., Kong, M. G., & Zimmermann, J. L. (2009). Focus on plasma medicine. New Journal of Physics, 11(11), 115011. https://doi.org/10.1088/1367-2630/11/11/115011
- Whittaker, A. G., Graham, E. M., Baxter, R. L., Jones, A. C., Richardson, P. R., Meek, G., … & Timoshkin, I. V. (2004). Plasma cleaning of dental instruments. Journal of Hospital Infection, 56(1), 37-41. https://doi.org/10.1016/j.jhin.2003.09.019
- Moisan, M., Barbeau, J., Moreau, S., Pelletier, J., Tabrizian, M., & Yahia, L. H. (2001). Low-temperature sterilization using gas plasmas: a review of the experiments and an analysis of the inactivation mechanisms. International journal of pharmaceutics, 226(1-2), 1-21. https://doi.org/10.1016/S0378-5173(01)00752-9
- von Woedtke, T., Reuter, S., Masur, K., & Weltmann, K. D. (2013). Plasmas for medicine. Physics Reports, 530(4), 291-320. https://doi.org/10.1016/j.physrep.2013.05.005
- Alkawareek, M. Y., Gorman, S. P., Graham, W. G., & Gilmore, B. F. (2014). Potential cellular targets and antibacterial efficacy of atmospheric pressure non-thermal plasma. International journal of antimicrobial agents, 43(2), 154-160. https://doi.org/10.1016/j.ijantimicag.2013.08.022
- Ghaffari, A., Miller, C. C., McMullin, B., & Ghahary, A. (2006). Potential application of gaseous nitric oxide as a topical antimicrobial agent. Nitric Oxide, 14(1), 21-29. https://doi.org/10.1016/j.niox.2005.08.003
- Privat-Maldonado, A., Schmidt, A., Lin, A., Weltmann, K. D., Wende, K., Bogaerts, A., & Bekeschus, S. (2019). ROS from physical plasmas: redox chemistry for biomedical therapy. Oxidative medicine and cellular longevity, 2019, 9062098. https://doi.org/10.1155/2019/9062098
- Zhu, W., Lee, S. J., Castro, N. J., Yan, D., Keidar, M., & Zhang, L. G. (2016). Synergistic effect of cold atmospheric plasma and drug loaded core-shell nanoparticles on inhibiting breast cancer cell growth. Scientific reports, 6, 21974. https://doi.org/10.1038/srep21974
- Leduc, M., Guay, D., Leask, R. L., & Coulombe, S. (2009). Cell permeabilization using a non-thermal plasma. New Journal of Physics, 11(11), 115021. https://doi.org/10.1088/1367-2630/11/11/115021
- Moncada, S., & Higgs, E. A. (1993). The L-arginine-nitric oxide pathway. New England Journal of Medicine, 329(27), 2002-2012. https://doi.org/10.1056/NEJM199312303292706
- Choi, M.-C., Cheung, K.-K., Li, X., & Cheing, G. L.-Y. (2016). Pulsed electromagnetic field (PEMF) promotes collagen fibre deposition associated with increased myofibroblast population in the early healing phase of diabetic wound. Archives of Dermatological Research, 308, 21–29. https://doi.org/10.1007/s00403-015-1604-9
- Brehmer, F., Haenssle, H. A., Daeschlein, G., Ahmed, R., Pfeiffer, S., Görlitz, A., … & Emmert, S. (2015). Alleviation of chronic venous leg ulcers with a hand-held dielectric barrier discharge plasma generator (PlasmaDerm® VU-2010): results of a monocentric, two-armed, open, prospective, randomized and controlled trial (NCT01415622). Journal of the European Academy of Dermatology and Venereology, 29(1), 148-155. https://doi.org/10.1111/jdv.12490
- Kubinova, S., Zaviskova, K., Uherkova, L., Zablotskii, V., Churpita, O., Lunov, O., & Dejneka, A. (2017). Non-thermal air plasma promotes the healing of acute skin wounds in rats. Scientific reports, 7(1), 45183. https://doi.org/10.1038/srep45183
- Haertel, B., von Woedtke, T., Weltmann, K. D., & Lindequist, U. (2014). Non-thermal atmospheric-pressure plasma possible application in wound healing. Biomolecules & therapeutics, 22(6), 477-490. https://doi.org/10.4062/biomolther.2014.105
- Shashurin, A., Keidar, M., Bronnikov, S., Jurjus, R. A., & Stepp, M. A. (2008). Living tissue under treatment of cold plasma atmospheric jet. Applied Physics Letters, 93(18), 181501. https://doi.org/10.1063/1.3020223
- Kalghatgi, S., Kelly, C. M., Cerchar, E., Torabi, B., Alekseev, O., Fridman, A., … & Azizkhan-Clifford, J. (2011). Effects of non-thermal plasma on mammalian cells. PloS one, 6(1), e16270. https://doi.org/10.1371/journal.pone.0016270
- Cha, S., & Park, Y. S. (2014). Plasma in dentistry. Clinical Plasma Medicine, 2(1), 4-10. https://doi.org/10.1016/j.cpme.2014.04.002
- Witte, M. B., & Barbul, A. (2002). Role of nitric oxide in wound repair. The American Journal of Surgery, 183(4), 406-412. https://doi.org/10.1016/S0002-9610(02)00815-2
- Thirumdas, R., Sarangapani, C., & Annapure, U. S. (2015). Cold plasma: A novel non-thermal technology for food processing. Food Biophysics, 10(1), 1-11. https://doi.org/10.1007/s11483-014-9382-z
- Randeniya, L. K., & de Groot, G. J. J. B. (2015). Non-thermal plasma treatment of agricultural seeds for stimulation of germination, removal of surface contamination and other benefits: A review. Plasma Processes and Polymers, 12(7), 608-623. https://doi.org/10.1002/ppap.201500042
- Bormashenko, E., Grynyov, R., Bormashenko, Y., & Drori, E. (2012). Cold radiofrequency plasma treatment modifies wettability and germination speed of plant seeds. Scientific Reports, 2, 741. https://doi.org/10.1038/srep00741
- Ling, L., Jiafeng, J., Jiangang, L., Minchong, S., Xin, H., Hanliang, S., & Yuanhua, D. (2014). Effects of cold plasma treatment on seed germination and seedling growth of soybean. Scientific Reports, 4, 5859. https://doi.org/10.1038/srep05859
- Ito, M., Oh, J. S., Ohta, T., Shiratani, M., & Hori, M. (2018). Current status and future prospects of agricultural applications using atmospheric-pressure plasma technologies. Plasma Processes and Polymers, 15(2), 1700073. https://doi.org/10.1002/ppap.201700073
- Mitra, A., Li, Y. F., Klämpfl, T. G., Shimizu, T., Jeon, J., Morfill, G. E., & Zimmermann, J. L. (2014). Inactivation of surface-borne microorganisms and increased germination of seed specimen by cold atmospheric plasma. Food and Bioprocess Technology, 7(3), 645-653. https://doi.org/10.1007/s11947-013-1126-4
- Zahoranová, A., Henselová, M., Hudecová, D., Kaliňáková, B., Kováčik, D., Medvecká, V., & Černák, M. (2016). Effect of cold atmospheric pressure plasma on the wheat seedlings vigor and on the inactivation of microorganisms on the seeds surface. Plasma Chemistry and Plasma Processing, 36(2), 397-414. https://doi.org/10.1007/s11090-015-9684-z
- Kumar, P., Patra, A. K., Mandal, G. P., Samanta, I., & Pradhan, S. (2017). Effect of black cumin seeds on growth performance, nutrient utilization, immunity, gut health and nitrogen excretion in broiler chickens. Journal of the Science of Food and Agriculture, 97(11), 3742–3751. https://doi.org/10.1002/jsfa.8237
- Puligundla, P., Kim, J. W., & Mok, C. (2017). Effect of corona discharge plasma jet treatment on decontamination and sprouting of rapeseed (Brassica napus L.) seeds. Food Control, 71, 376-382. https://doi.org/10.1016/j.foodcont.2016.07.021
- Li, L., Jiang, J., Li, J., Shen, M., He, X., Shao, H., & Dong, Y. (2014). Effects of cold plasma treatment on seed germination and seedling growth of soybean. Scientific Reports, 4, 5859. https://doi.org/10.1038/srep05859
- Misra, N. N., Yadav, B., Roopesh, M. S., & Jo, C. (2019). Cold plasma for effective fungal and mycotoxin control in foods: Mechanisms, inactivation effects, and applications. Comprehensive Reviews in Food Science and Food Safety, 18(1), 106-120. https://doi.org/10.1111/1541-4337.12398
- Lacombe, A., Niemira, B. A., Gurtler, J. B., Fan, X., Sites, J., Boyd, G., & Chen, H. (2015). Atmospheric cold plasma inactivation of aerobic microorganisms on blueberries and effects on quality attributes. Food Microbiology, 46, 479-484. https://doi.org/10.1016/j.fm.2014.09.010
- Thirumdas, R., Kothakota, A., Annapure, U., Siliveru, K., Blundell, R., Gatt, R., & Valdramidis, V. P. (2018). Plasma activated water (PAW): Chemistry, physico-chemical properties, applications in food and agriculture. Trends in Food Science & Technology, 77, 21-31. https://doi.org/10.1016/j.tifs.2018.05.007
- Zhou, R., Zhou, R., Zhang, X., Zhuang, J., Yang, S., Bazaka, K., & Ostrikov, K. (2016). Effects of atmospheric-pressure N2, He, air, and O2 microplasmas on mung bean seed germination and seedling growth. Scientific Reports, 6, 32603. https://doi.org/10.1038/srep32603
- Xiang, Q., Liu, X., Li, J., Liu, S., Zhang, H., & Bai, Y. (2018). Effects of plasma-activated water on microbial growth and quality of fresh-cut apples. Innovative Food Science & Emerging Technologies, 49, 217-225. https://doi.org/10.1016/j.ifset.2018.09.003
- Zhang, Q., Ma, R., Tian, Y., Su, B., Wang, K., Yu, S., … & Fang, J. (2016). Sterilization efficiency of a novel electrochemical disinfectant against Staphylococcus aureus. Environmental Science & Technology, 50(6), 3184-3192. https://doi.org/10.1021/acs.est.5b05108
- Laurita, R., Barbieri, D., Gherardi, M., Colombo, V., & Lukes, P. (2015). Chemical analysis of reactive species and antimicrobial activity of water treated by nanosecond pulsed DBD air plasma. Clinical Plasma Medicine, 3(2), 53-61. https://doi.org/10.1016/j.cpme.2015.10.001
- Sivachandiran, L., & Khacef, A. (2017). Enhanced seed germination and plant growth by atmospheric pressure cold air plasma: combined effect of seed and water treatment. RSC Advances, 7(4), 1822-1832. https://doi.org/10.1039/C6RA24762H
- Jiang, J., Lu, Y., Li, J., Li, L., He, X., Shao, H., & Dong, Y. (2014). Effect of seed treatment by cold plasma on the resistance of tomato to Ralstonia solanacearum (bacterial wilt). PloS One, 9(5), e97753. https://doi.org/10.1371/journal.pone.0097753
- Besson-Bard, A., Pugin, A., & Wendehenne, D. (2008). New insights into nitric oxide signaling in plants. Annual Review of Plant Biology, 59, 21-39. https://doi.org/10.1146/annurev.arplant.59.032607.092830
- Dowling, J. P., Scalora, M., Bloemer, M. J., & Bowden, C. M. (1994). The photonic band edge laser: A new approach to gain enhancement. Journal of Applied Physics, 75(4), 1896-1899. https://doi.org/10.1063/1.356336
- Chu, P. K., Chen, J. Y., Wang, L. P., & Huang, N. (2002). Plasma-surface modification of biomaterials. Materials Science and Engineering: R: Reports, 36(5-6), 143-206. https://doi.org/10.1016/S0927-796X(02)00004-9
- Deng, X., Shi, J., & Kong, M. G. (2006). Physical mechanisms of inactivation of Bacillus subtilis spores using cold atmospheric plasmas. IEEE Transactions on Plasma Science, 34(4), 1310-1316. https://doi.org/10.1109/TPS.2006.877739
- Garratty, G. (1994). Immunobiology of Transfusion Medicine (1st ed.). CRC Press. https://doi.org/10.1201/9781482293494
- Jiang, B., Zheng, J., Qiu, S., Wu, M., Zhang, Q., Yan, Z., & Xue, Q. (2014). Review on electrical discharge plasma technology for wastewater remediation. Chemical Engineering Journal, 236, 348-368. https://doi.org/10.1016/j.cej.2013.09.090
- Mizuno, A. (2007). Industrial applications of atmospheric non-thermal plasma in environmental remediation. Plasma Physics and Controlled Fusion, 49(5A), A1. https://doi.org/10.1088/0741-3335/49/5A/S01
- Shenton, M. J., & Stevens, G. C. (2001). Surface modification of polymer surfaces: atmospheric plasma versus vacuum plasma treatments. Journal of Physics D: Applied Physics, 34(18), 2761. https://doi.org/10.1088/0022-3727/34/18/308
Related articles
Made in USA
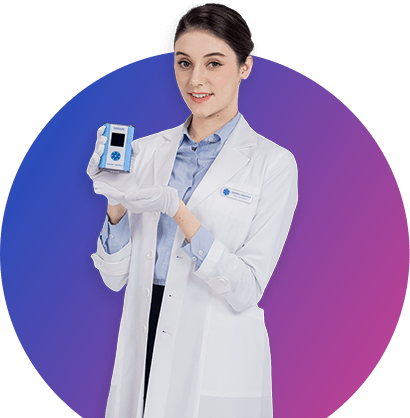