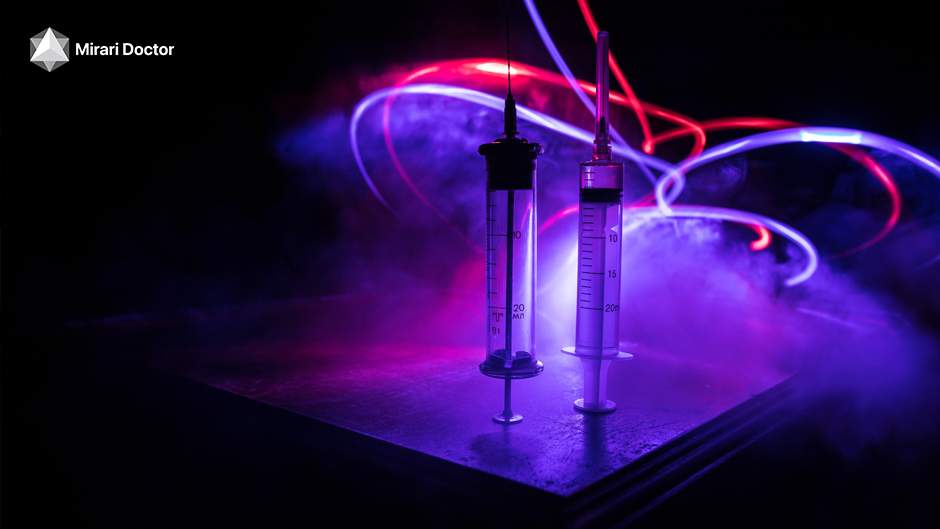
You might be interested
Cold plasma technology is an advanced and revolutionary field gaining traction due to its broad spectrum of applications across different industries, including food safety, medicine, aerospace, automotive, and environmental management. Unlike hot plasmas, temperature in cold plasmas remains close to room temperature, which means it can deactivate bacteria, viruses, and other pathogens without causing damage to the surfaces being treated. This unique feature is what makes cold plasma technology so versatile and effective.
One notable innovation in this field is the Mirari Cold Plasma device, developed by General Vibronics. This handheld device utilizes groundbreaking technology that harnesses the power of nitric oxide (NO) to create a unique form of non-invasive cold plasma. The Mirari Cold Plasma System is approved by the Thai FDA and Vietnam MOH for specific uses, showcasing how cold plasma technology is being translated into practical, accessible tools for medical applications.
By understanding the different types of cold plasma systems and the challenges faced in their implementation, one can better appreciate the potential and constraints of this cutting-edge technology. Join us as we delve into the various systems that make up cold plasma technology, their applications, and examine the pertinent challenges they face in commercialization and practical use.
Types of cold plasma systems
There’s a vast range of cold plasma systems, each with specific configurations and applications. The most common types include Dielectric Barrier Discharge (DBD) systems, Atmospheric Pressure Plasma Jet (APPJ) systems, Microwave Plasma systems, Radiofrequency Plasma systems, and Electrode-less Plasma systems. Each type has unique characteristics and operational principles, akin to different musical instruments in an orchestra, contributing distinct notes to the collective symphony of technological advancement.
Dielectric Barrier Discharge (DBD) Systems
Dielectric Barrier Discharge (DBD) systems stand at the forefront of cold plasma technology. First developed in the mid-19th century, these systems have evolved to become a staple in various industries due to their efficacy and versatility.
Atmospheric Pressure Plasma Jet (APPJ) Systems
Atmospheric Pressure Plasma Jet (APPJ) systems offer another groundbreaking approach in cold plasma technology. They are likened to precision instruments in a skilled surgeon’s toolkit, delivering targeted plasma streams for specific applications.
- Operation and Design:
- Structure: APPJ systems utilize a flowing gas (e.g., argon, helium) that is ionized to form a plasma jet. Construction typically involves electrodes encased within dielectric tubes and a nozzle to guide the plasma flow. The Mirari Cold Plasma device is an example of an APPJ system, providing targeted cold plasma application in a handheld format.
- Mechanism: Through a dielectric barrier discharge mechanism, APPJ systems ionize the flowing gas, creating a stable plasma jet that extends beyond the dielectric surface.
- Key Features:
- Non-Thermal: Operating at temperatures below 40°C, APPJ systems are suitable for treating biological tissues without causing thermal damage.
- Reactive Species Generation: They efficiently generate reactive species like oxygen radicals and nitrogen derivatives, crucial for applications requiring sterilization and surface treatment.
- Portability: APPJ devices are compact and easy to handle, making them ideal for precise, spot treatments.
- Applications:
- Medical Field: Extensively used in wound healing, cancer treatments by targeting and breaking down tumor cells, and dental applications for sterilization.
- Surface Sterilization: Employed in sterilizing surfaces in food processing and medical environments, effectively reducing contamination risks.
- Textiles: Enhancing the wettability and dye uptake of fabrics.
- Pros and Cons:
- Pros: High precision in targeting specific areas, effective in generating significant quantities of reactive species, user-friendly design.
- Cons: Potential limitations in treating large surfaces uniformly, dependency on the type of gases used which may impact costs and operational feasibility.
Microwave Plasma Systems
Microwave Plasma Systems bring another dimension to the cold plasma realm, endeavoring to harness the energy efficiency of microwaves to generate plasma. Much like the transition from traditional cooking to microwave ovens, this technology signifies a leap in operational efficiency and speed.
- Operation and Design:
- Structure: Microwave plasma systems use microwave radiation to ionize gases within a chamber. They typically involve a microwave generator, waveguides, and a plasma reactor.
- Mechanism: Microwaves ionize gas molecules by exciting them with electromagnetic radiation, leading to plasma formation.
- Key Features:
- High Plasma Density: Capable of producing highly dense plasma, leading to more efficient reactions.
- Controlled Environment: These systems can operate in varied environmental conditions and are customizable for different applications.
- Minimal Heat Generation: They maintain low operational temperatures, suitable for sensitive applications.
- Applications:
- Material Processing: Utilized in the deposition of thin films and coatings, vital for electronics and semiconductor industries.
- Biological Decontamination: Effective in treating surfaces for microbial inactivation in medical and food processing facilities.
- Environmental Remediation: Applied in breaking down pollutants for air and water purification.
- Pros and Cons:
- Pros: High-density plasma for more effective treatments, operational flexibility, and minimal heat impact.
- Cons: High initial setup costs, complexity in design and maintenance, energy consumption considerations.
Radiofrequency Plasma Systems
Radiofrequency (RF) plasma systems use radiofrequency waves to generate plasma, making them versatile and valuable in a variety of settings. Envisioning RF plasma systems is akin to tuning into different radio frequencies to capture diverse broadcasts, each serving unique constituents.
- Operation and Design:
- Structure: These systems operate typically at frequencies around 13.56 MHz and involve electrodes or coils to generate and sustain plasma.
- Mechanism: RF waves ionize the gas in the chamber, creating plasma which can interact with materials positioned inside.
- Types of RF Configurations:Capacitively Coupled Plasma (CCP):
- Structure: Two parallel electrodes create an electric field.
- Application: Used in processes requiring uniform plasma, such as semiconductor manufacturing.
Inductively Coupled Plasma (ICP):
- Structure: Coils generate a magnetic field that sustains the plasma.
- Application: Suitable for high-density plasma generation, beneficial for material etching and deposition processes.
- Applications:
- Food Processing: Microbial decontamination of food surfaces to enhance safety and shelf life.
- Material Modification: Surface treatments to improve adhesion, hydrophobicity, or antibacterial properties.
- Sterilization: Effective sterilization of medical instruments and devices.
- Pros and Cons:
- Pros: Capability to create uniform plasma over large areas, versatile application potential, high-density plasma suitable for intricate material treatments.
- Cons: Complex control mechanisms required, potential high operational costs, and energy consumption.
Electrode-less Plasma Systems
Electrode-less Plasma Systems represent an innovative leap in cold plasma technology, similar to going wireless in telecommunications. By eliminating electrodes, these systems avoid contamination and enhance the purity and efficiency of plasma generation.
- Operation and Design:
- Structure: These systems employ methods like microwave or RF waves to excite gases without direct electrode contact.
- Mechanism: Microwaves or RF waves interact with the gas, leading to ionization and plasma formation within the excitation field.
- Key Features:
- No Electrode Contamination: Reduces the risks of contamination from electrode materials.
- Versatility: Operate effectively at atmospheric pressure, enhancing adaptability for various applications.
- Lower Operational Costs: Elimination of electrodes reduces maintenance and replacement costs.
- Applications:
- Surface Modification: Widely used in modifying surface properties for increased biocompatibility in medical devices.
- Food Processing: Microbial inactivation and enhancing food safety without affecting product quality.
- Environmental Cleanup: Applied in the treatment of contaminants in air and water.
- Pros and Cons:
- Pros: Reduced contamination risk, flexible operation, cost-effective maintenance.
- Cons: Potential limitations in power delivery efficiency, complex design considerations for consistent plasma generation.
Applications of cold plasma technology
Cold plasma technology’s multifaceted applications span various industries, much like a well-composed symphony with multiple sections contributing to the overall harmony. Key areas include food safety, surface modification, wastewater treatment, medical applications, and agricultural enhancements.
Food safety and preservation
Food safety and preservation are critical concerns globally, and cold plasma technology offers promising solutions without the drawbacks associated with traditional methods. Foods treated with cold plasma undergo minimal thermal damage, preserving nutritional quality and sensory attributes.
- Microbial Decontamination:
- Purpose: Primarily to ensure food safety by reducing or eliminating microbial load.
- Effectiveness: Cold plasma has proven effective in inactivating bacteria, fungi, and spores, ensuring hygiene in ready-to-eat (RTE) foods.
- Food Packaging:
- Surface Treatment: Enhancing microbial safety of packaging materials by reducing bacterial adhesion.
- Barrier Properties: Improving barrier properties to extend the shelf life and maintain food quality.
- Juice Preservation:
- Effect on Juices: Cold plasma extends the shelf life of juices without adversely affecting taste or nutritional content.
- Case Studies: Research indicates significantly longer refrigerated shelf life for cold plasma-treated juices.
- Surface Decontamination:
- Food Processing: Cleaning and sterilizing food contact surfaces to reduce cross-contamination risks.
- Efficiency: Highly effective in maintaining hygiene in processing environments.
- Allergen and Pesticide Reduction:
- Degradation: Cold plasma can degrade allergens and pesticide residues, ensuring the safety of fresh produce and other foods.
- Benefit: Critical for foods where residual chemicals are a significant concern, such as organic products.
Surface modification of materials
Cold plasma technology is extensively used in the surface modification of materials, akin to a master artist refining the texture and composition of their canvas.
- Surface Chemistry and Topography:
- Functionalization: Introducing functional groups to improve chemical reactivity and adhesion properties, vital in coating and bonding processes.
- Applications: In sectors like aerospace, automotive, and biomedical engineering, where surface properties are crucial for performance.
- Wide Range of Applications:
- Biomedical Field: Modifying polymer surfaces to promote cell adhesion and biocompatibility, essential for implants and prosthetics.
- Electronics: Enhancing surface properties for improved performance in electronic components.
- Environmental Benefits:
- Energy Efficiency: Typically low-temperature processes minimize energy consumption.
- Sustainability: Allows for the treatment of heat-sensitive materials without environmental damage.
Wastewater treatment
The scarcity and contamination of water resources call for advanced treatment methods, and cold plasma technology presents a novel solution.
- Advanced Oxidation Processes (AOP):
- Reactive Species Generation: Cold plasma generates reactive species (e.g., OH, O, H) capable of oxidizing and decomposing organic pollutants.
- Effectiveness: More effective than traditional methods against emerging contaminants.
- Inactivation of Pathogens:
- Disinfection: Proven effective in inactivating pathogens, enhancing public health safety standards.
- Recent Findings: Studies show effectiveness against viruses like SARS-CoV-2, underlining its potential in maintaining hygiene.
- Operational Flexibility:
- Types of Discharges: Employing DBD, plasma jets, and pulsed corona discharge to treat various contaminants.
- Adaptability: Allows custom solutions for different treatment goals and contaminant types.
Medical applications: sterilization and wound healing
Cold atmospheric plasma (CAP) technology excels in medical applications, predominantly in the sterilization of devices and wound healing. Devices like the Mirari Cold Plasma system are at the forefront of making this technology accessible for clinical use.
- Sterilization:
- Effectiveness: CAP can inactivate a range of pathogens by generating reactive species that damage microbial cells.
- Application: Widely adopted for sterilizing medical and dental devices without residual chemicals or heat damage. Handheld devices like the Mirari system allow for targeted sterilization.
- Wound Healing:
- Bacterial Load Reduction: CAP efficiently reduces bacterial load in chronic wounds.
- Tissue Regeneration: Stimulates tissue repair by promoting angiogenesis and collagen synthesis. The Mirari Cold Plasma device’s non-invasive application allows for direct treatment of wounds.
Agricultural enhancements
Cold plasma is showing promise in agriculture, from pathogen control to improving seed vitality.
- Pathogen Control:
- Disinfection: CAP effectively disinfects seeds, soil, and agricultural products.
- Benefit: Maintains crop health and improves yields.
- Seed Treatment:
- Germination Rates: Plasma treatment enhances germination rates and seedling vigor.
- Growth Boost: Stimulates growth factors in seeds, leading to healthier plants.
- Plant Growth Enhancement:
- Plasma-Activated Water (PAW): Improves nutrient absorption and stress tolerance in plants.
- Application: Used in irrigation to enhance overall plant health.
Mechanisms of cold plasma interaction
Understanding the mechanisms of cold plasma interaction with materials and biological entities is crucial for optimizing its applications. Cold plasma generates reactive species, each playing specific roles in different interaction mechanisms.
Reactive species generated
Cold plasma treatment results in the formation of various reactive species, pivotal in its effectiveness.
- Reactive Species Examples:
- Reactive Oxygen Species (ROS): Includes .OH, H2O2, and O3, potent oxidizers responsible for altering microbial cells and food biomolecules.
- Reactive Nitrogen Species (RNS): Nitric oxide (NO) and nitrogen dioxide (NO2), contributing to microbial inactivation and biochemical modifications.
- Electromagnetic Radiation: Emission of UV radiation inducing photochemical reactions.
Mechanisms for microbial inactivation
Cold plasma inactivates microorganisms through a combination of radical interactions and physical disruptions.
- Reactive Species Production:
- Role of RONS: Key players in inducing oxidative stress and microbicidal effects.
- Effect on Microorganisms: Disrupting cell membranes, proteins, and nucleic acids.
- Cell Membrane Damage:
- Mechanics: Causes lipid peroxidation, leading to loss of membrane integrity and cell death.
- Target: Particularly effective against Gram-negative bacteria.
- Intracellular Damage:
- Penetration: Cold plasma permeates into cells, disrupting DNA and metabolic processes.
- Outcome: Induces necrosis or apoptosis, depending on exposure levels.
Plasma-induced chemical reactions
The reactive species generated by cold plasma initiate various chemical reactions in treated materials.
- Oxidation Reactions:
- Species Involved: ROS like hydroxyl radicals and ozone interact with food and material biomolecules, causing oxidation.
- Result: Alters nutritional profiles and sensory attributes.
- Modification of Biomolecules:
- Impact on Food: Cold plasma induces structural changes in proteins, carbohydrates, and lipids.
- Enhancements: Improves functional properties beneficial for food processing.
Challenges in cold plasma technology
While the advantages of cold plasma technology are manifold, its broader implementation is stymied by several challenges.
Regulatory and safety concerns
The prevailing regulatory status and safety concerns present substantial barriers.
- Regulatory Landscape:
- Inconsistencies: Lack of definitive guidelines across countries hampers commercialization.
- Challenges: Producers may be reluctant to invest without clear regulatory support.
- Safety Issues:
- Residual Compounds: Health risks from reactive species residues on food products remain a concern.
- Research Needs: More studies are required to ascertain long-term effects.
Economic viability and cost-effectiveness
Ensuring economic viability and maintaining cost-effectiveness are critical for adopting cold plasma technology on a large scale.
- Initial Investment:
- High Costs: Significant upfront costs create barriers, especially for small enterprises.
- Operational Expenses: Continuous operational costs must be minimized for sustainable use.
- Scalability Challenges:
- Consistency: Maintaining consistent treatment outcomes during scale-up is complex.
- System Variation: Different systems (low-pressure vs. atmospheric pressure) have distinct cost and efficiency implications.
Scale-up and commercialization issues
Scaling cold plasma technology from laboratory to industrial levels involves overcoming various obstacles.
- System Designs:
- Variability: Diverse system designs complicate standardization and repeatability.
- Efficiency: Optimizing systems for large-scale use while managing costs is crucial.
- Commercial Viability:
- Capital Investment: High initial investment and technology complexity can deter large-scale adoption.
- Practical Considerations: Balancing cost, efficiency, and scalability remains a significant hurdle.
Knowledge gaps in mechanistic understanding
There are notable gaps in understanding the specific mechanisms underpinning cold plasma interactions.
- Mechanistic Insights:
- Research Needs: More comprehensive studies on the interactions and specific mechanisms are necessary.
- Optimization: Understanding these mechanisms will aid in optimizing cold plasma applications.
Material compatibility and longevity
Ensuring compatibility and longevity of materials exposed to cold plasma remains a challenge.
- Material Durability: -1. Material Longevity:
- Exposure Effects: Prolonged exposure to reactive species may degrade materials used in food processing equipment.
- Research Needs: Investigating durable materials that can withstand continuous exposure while maintaining functionality is vital.
- Compatibility Factors:
- Diverse Materials: Different materials respond uniquely to cold plasma treatment, necessitating tailored solutions for each type.
- Safety and Efficiency: Ensuring materials are both effective for plasma applications and safe for prolonged use is essential for widespread adoption.
Future directions in cold plasma research
Advancements in cold plasma technology continue to emerge, focusing not just on refining systems and enhancing performance but also on integrating the technology with other processes.
Innovations in system design
Future research is geared towards significant innovations in cold plasma system design, improving both efficiency and versatility.
Enhanced performance metrics
Research aims to enhance the performance metrics of cold plasma systems, improving their efficiency, accuracy, and reliability.
- Advanced Characterization Techniques:
- Mass Spectrometry and Optical Emission Spectroscopy: Using these techniques to analyze plasma properties and optimize treatment outcomes.
- Real-Time Monitoring: Implementing systems for real-time monitoring to adjust parameters dynamically for better precision and control.
- Data-Driven Optimization:
- Machine Learning: Utilizing AI and machine learning to predict and enhance treatment outcomes by optimizing operational parameters.
- Predictive Control: Developing predictive models to forecast the effects of cold plasma on different materials or biological systems.
- Interdisciplinary Collaboration:
- Cross-Field Initiatives: Encouraging collaboration among physicists, biologists, engineers, and industry experts to achieve more comprehensive and effective solutions.
For those interested in learning more about cutting-edge CAP technologies like the Mirari Cold Plasma device, visit the Mirari Doctor website at miraridoctor.com. As the field evolves, staying informed about the latest innovations will be key to harnessing the full potential of cold plasma in medicine and other applications.
Expanded applications across industries
Cold plasma technology’s potential applications are expanding across various industries, from healthcare to agriculture.
- Healthcare:
- Wound Healing: Improved protocols for using cold plasma in wound disinfection and tissue regeneration. Devices like the Mirari Cold Plasma system are leading the way in making this technology accessible for clinical use.
- Cancer Treatment: Cold plasma’s potential in breaking down tumor cells while sparing healthy tissue.
- Dental Applications: Enhanced methods for sterilizing dental tools and surfaces.
- Food Industry:
- Safe Preservation: Developing systems for applying cold plasma to maintain food safety and extend shelf life without compromising quality.
- Enhancing Packaging: Improving the microbial safety and barrier properties of packaging materials.
- Agriculture:
- Soil and Seed Treatment: Innovating methods to use cold plasma for pathogen control and improving crop vitality.
- Plant Growth Enhancement: Employing plasma-activated water to boost nutrient absorption and stress tolerance.
- Materials Science:
- Surface Engineering: Refining techniques for surface modification to enhance properties like adhesion and hydrophobicity.
- Environmental Applications: Innovations in treating pollutants in air and water through cold plasma processes.
Technological integration with other processes
Integrating cold plasma technology with other modalities promises to expand its application and effectiveness further.
- Multimodal Approaches:
- Combination Therapies: Integrating cold plasma with phototherapy, ultrasound, or other treatments to enhance therapeutic outcomes.
- Synergistic Effects: Exploring how combined treatments can work together to target cells more effectively, such as using cold plasma with chemotherapy in cancer treatment.
- Continuous Processing Systems:
- Food Processing: Developing systems for continuous processing of food products, incorporating cold plasma to enhance safety and quality.
- Industrial Integration: Embedding cold plasma systems into existing industrial processes to improve efficiency and reduce contamination.
- Standardization and Protocols:
- Unified Standards: Creating standardized protocols to ensure consistent and effective application across different industries.
- Training Programs: Developing training programs to educate operators on the safe and efficient use of cold plasma technologies.
Conclusion
Cold plasma technology represents a frontier of innovation across multiple industries, offering promising solutions in areas such as food safety, medical treatments, agricultural enhancements, and environmental management. However, to fully realize its potential, significant challenges must be addressed, including regulatory hurdles, economic viability, scalability, and material compatibility. Advancements in system design, data-driven optimization, and interdisciplinary collaboration are key to overcoming these obstacles and expanding the applications of cold plasma technology.
Devices like the Mirari Cold Plasma system, approved by regulatory bodies like the Thai FDA and Vietnam MOH for specific uses, are helping translate the promise of this technology into real-world applications, opening up exciting new possibilities in fields like medicine and beyond. The continued dedication to innovation and overcoming existing challenges will be crucial in harnessing the full power of this versatile and effective technology.
As cold plasma technology advances, its potential to transform industries and improve global standards in food safety, healthcare, environmental sustainability, and beyond becomes increasingly apparent. The future research directions highlighted in this article emphasize the need for ongoing exploration and development to enhance cold plasma systems’ efficiency and applicability. By focusing on improved performance metrics, expanded applications, and technological integration, this field can continue to grow and provide revolutionary benefits across various sectors.
Related articles
Made in USA
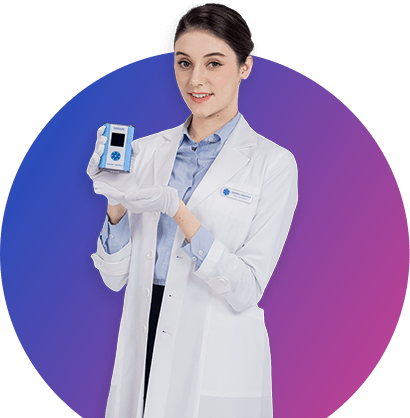